ApoE – functionalization of nanoparticles for targeted brain delivery – a feasible method for polyplexes?
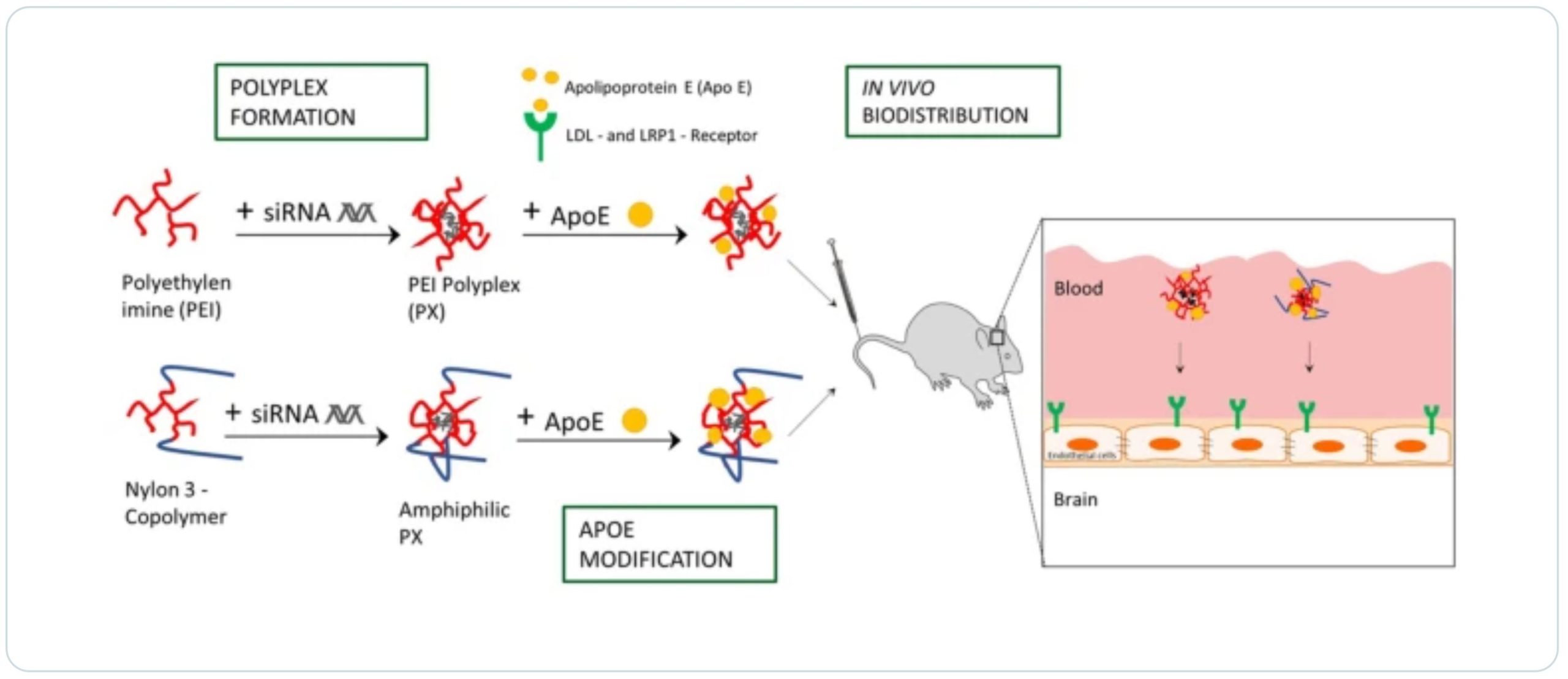
Abstract
The blood–brain barrier (BBB) poses a major obstacle in the treatment of all types of central nervous system (CNS) diseases. Small interfering RNA (siRNA) offers in principle a promising therapeutic approach by downregulating disease-related genes via RNA interference. However, the BBB is a formidable barrier for macromolecules such as nucleic acids. In an effort to develop a brain-targeted strategy for siRNA delivery systems formed by electrostatic interactions with cationic polymers (polyplexes (PXs)), we investigated the suitability of the well-known surfactant-based approach for Apolipoprotein E (ApoE)-functionalization of nanoparticles (NPs). The aim of this present work was to investigate if ApoE coating of siRNA PXs formed with cationic branched 25-kDa poly(ethyleneimine) (b-PEI) and nylon-3 polymers without or after precoating with polysorbate 80 (PS 80) would promote successful delivery across the BBB. We utilized highly hydrophobic NM0.2/CP0.8 nylon-3 polymers to evaluate the effects of hydrophobic cyclopentyl (CP) subunits on ApoE binding efficacy and observed successful ApoE binding with and without PS 80 precoating to the nylon-3 but not the PEI polyplexes. Accordingly, ApoE-coated nylon-3 polyplexes showed significantly increased uptake and gene silencing in U87 glioma cells but no benefit in vivo. In conclusion, further optimization of ApoE-functionalized polyplexes and more sophisticated in vitro models are required to achieve more successful in vitro–in vivo translation in future approaches.
Introduction
The ability of therapeutic agents to reach their target sites in the central nervous system (CNS) is very limited due to the strong protective function of the blood–brain barrier (BBB). However, according to the World Health Organization (WHO), approximately 20% of all humans suffer from CNS disorders such as depression, Parkinson’s disease, Alzheimer’s disease, dementia, epilepsy, stroke, cerebral cancer, or CNS-relevant metabolic diseases. According to recent estimates, the number of people suffering from CNS diseases worldwide will increase significantly in coming decades due to tremendous population growth, increasing life expectancy and in addition, due to risk factors such as obesity, smoking, high blood pressure, or diabetes. Therefore, the development of highly efficient, safe, and targeted pharmaceutical systems that are able to overcome the BBB is urgently needed in order to exert therapeutic effects in disease-specific regions of the brain. Macromolecular drugs, such as proteins, peptides, or nucleic acids, bear a very high potential to open up new perspectives for the treatment of CNS diseases. Less than 2% of small molecule drugs are able to cross the barrier to reach their target sides in the CNS [1]. When it comes to macromolecular drugs, the barrier is completely impermeable due to their high molecular weight [2]. For an extensive review covering transport routes across the BBB, the reader is referred to [3].
A wide range of macromolecular drugs is embedded into nanocarriers to make them stable, safe, and efficient drugs after administration into the human body. In an effort to design efficient nanocarriers that can enable controlled and targeted drug delivery into the brain, a promising option is to decorate the nanocarriers with ligands that can interact with specific binding sites of the BBB with subsequent internalization of the particles via receptor-mediated transcytosis (RMT) into the brain. Among the numerous potential targets at the BBB, the low-density lipoprotein (LDL) receptor family has been extensively investigated as highly efficient target for brain delivery in several studies using liposomes [4] or polymeric nanoparticles (NPs) [3] as drug carriers.
In early attempts, it was found out that some drugs that are commonly unable to cross the BBB can be transported across this barrier and exhibit pharmacological effects after intravenous injection by encapsulating them into poly (butyl cyanoacrylate) (PBCA) NPs. By using PBCA NPs as drug carriers, substances such as the hexapeptide dalargin [5,6,7,8,9,10,11], the dipeptide kytorphin [9], loperamide [12], tubocurarine [13], the NMDA receptor antagonist MRZ 2/576 [14], and doxorubicin [15,16,17] have already been successfully transported into the brain. More continuing studies have confirmed that the brain targeting effect can be further enhanced by precoating the PBCA NPs with surfactants such as PS 20, 40, 60, and 80 [18]. Following the identification of apolipoprotein E (ApoE) on the surface of PS-precoated PBCA NPs after incubation in human plasma [19], Kreuter et al. succeeded in confirming the involvement of plasma ApoE in the brain uptake mechanism of loperamide or dalargin-loaded PBCA NPs (both analgesics) after intravenous injection in mice by measuring the antinociceptive effects with the tail flick test [20].
Since then, numerous small molecule drugs, e.g., methotrexate [21] and cisplatin [22] for the treatment of cerebral cancer or tacrine [23, 24], rivastigmine [25] or rosmarinic acid [26] for treatment of Alzheimer’s disease, and peptide/protein drugs such as the nerve growth factor [27, 28] also for the treatment of Alzheimer’s disease or arylsulfatase A [29] for metachromatic leukodystrophy have been successfully delivered into the brain using PS-coated PBCA NPs. Subsequently, the ApoE targeting approach was successfully applied to several other matrix NPs, e.g., human and bovine serum albumin (HAS [30] and BSA [31]), poly(lactic acid) (PLA) [32, 33], and poly(lactic-co-glycolic acid) (PLGA) [34,35,36,37] NPs. Moreover, in addition to the surfactant-based approach, other types of ApoE functionalization of NPs have been investigated such as direct coating of NPs with ApoE, adsorption of ApoE onto NPs by poly(ethylene glycol) (PEG) modification, or covalent linkage of ApoE to NPs.
In an effort to investigate whether the already established brain delivery strategy for solid NPs is also suitable for dynamic systems such as polymer-small interference RNA (siRNA) polyelectrolyte complexes (polyplexes) formed by electrostatic interactions, we evaluated the suitability of the surfactant-based approach with PS 80. The application of siRNA is a promising therapeutic approach in particular for brain tumors due to its ability to downregulate glioma-related genes and to induce tumor growth inhibition, as recently demonstrated in vitro as well as in vivo experiments [38,39,40]. Since PSs were shown to operate as hydrophobic anchors for binding ApoE, the question arose whether hydrophobic modification of polymers could lead to enhanced ApoE binding without surfactant precoating, what could be beneficial due to concerns and contradictions regarding surfactants’ toxicity [41].
Therefore, besides branched poly(ethyleneimine) (b-PEI) polymers, this study utilized nylon-3 polymers with hydrophobic subunits derived from β-lactam cyclopentyl (CP) and cationic subunits derived from β-lactam without (“no”) methyl substitution (NM) with a subunit ratio of 1:4 (NM:CP), which have been previously investigated regarding efficacy of siRNA delivery into brain tumor cells [42]. Herein, we coated b-PEI and NM0.2/CP0.8 PXs with ApoE with and without PS 80 precoating and characterized the resulting PXs in terms of their physicochemical characteristics such as particle size, particle size distribution, and surface charge. Sodium dodecylsulfate polyacrylamide gel electrophoresis (SDS-PAGE) was established as a suitable method to verify bound ApoE on PEI and nylon-3 PXs by visualizing them with Comassie Brilliant Blue G staining. Furthermore, cell tolerability, cellular internalization ability, and gene knockdown efficiency of modified and unmodified PEI and nylon-3 PXs were evaluated by CellTiter-Blue® assay, flow cytometry, and real-time quantitative PCR (qPCR) using an LDL and LRP1 receptor expressing model cell line. Since we obtained very promising results in in vitro experiments particularly for ApoE-coated nylon-3 PXs, we first confirmed the hemocompatibility of polymers using a hemolysis assay and subsequently investigated their biodistribution in comparison to b-PEI PXs by measuring the radioactive signals of encapsulated 177Lu-labeled DTPA-modified siRNA in the major organs and the brain after intravenous injection in SWISS mice.
Download the full article as PDF here: ApoE—functionalization of nanoparticles for targeted brain delivery—a feasible method for polyplexes
or read it here
Materials
Branched poly(ethylene imine) (b-PEI) 25 kDa, apolipoprotein E (ApoE) from human plasma, HEPES (4-(2-hydroxyethyl)–1-piperazineethanesulfonic acid), Comassie Brilliant Blue G solution, sodium acetate, sodium chloride, sodium hydroxide, sodium hydrogen carbonate, Tween® 80, Dulbecco’s phosphate-buffered saline (PBS), Triton-X, arsenazo(III), yttrium(III) chloride, and for cell culture U87 cells (human glioblastoma astrocytoma), Eagle’s minimum essential medium (EMEM), fetal bovine serum (FBS), penicillin–streptomycin solution, trypsin–EDTA solution 0.25%, and dimethyl sulfoxide (DMSO) were purchased from Sigma-Aldrich (Taufkirchen, Germany). Novex™ 10% tris–glycine gel, PageRuler™ Plus Prestained Protein Ladder (10 to 250 kDA), Pierce™ Lane Marker Reducing Sample Buffer, and absolute ethanol were purchased from Thermo Fisher Scientific (Waltham, MA, USA). Gibco® trypan blue solution 0.4% in phosphate-buffered saline was obtained from FisherScientific (Hampton, New Hampshire, USA). Lipofectamin 2000 transfection reagent and AlexaFluor 488 (AF488) dye were purchased from Life Technologies (Carlsbad, California, USA). CellTiter-Blue® Cell Viability Assay was purchased from Promega (Madison, Wisconsin, USA). Rotiphorese®10 × SDS-PAGE buffer was obtained from Carl Roth (Karlsruhe, Germany), and mouse ApoE was purchased from Abexxa (Cambridge, UK). 2-(4-Isothiocyanatobenzyl) diethylenetriaminepentaacetic acid (p-SCN-Bn-DTPA) was purchased from Macrocyclics (Dallas, TX, USA) and Hs_GAPDH_1_SG and Hs_ACTB_2_SG QuantiTect primer assays were obtained from Qiagen (Venlo, Netherlands). Fresh human blood was obtained from Blutspendedienst des Bayerischen Roten Kreuzes (Munich, Germany), and EndolucinBeta-Lu3+ in aqueous 0.04 M HCl solution was purchased from ITG Isotope Technologies Garching GmbH (Garching, Germany).
Hartl, N., Gabold, B., Uhl, P. et al. ApoE—functionalization of nanoparticles for targeted brain delivery—a feasible method for polyplexes?. Drug Deliv. and Transl. Res. (2023). https://doi.org/10.1007/s13346-023-01482-w