Unlocking the Mystery of Glass Transition Temperatures – Explainer video by Pharma Drama

In this video Pharma Drama aka Prof. Simon Gaisford explains the Glass Transition Temperature (or Tg). He looks at the glass transition in easy-to-understand terms; what it is and what happens to a material to make a glass. The concept is highlighted with diagrams, video and an indecent amount of indomethacin.
See the video and read the full transcript below.
Read the full video transcript here:
Welcome to Pharma Drama, the channel where we look at the science of healthcare and healthcare products. Today we’re going to look at a peculiar behaviour unique to amorphous materials, and something I know many students find confusing, glass transitions. The glass transition is a fundamental characteristic of amorphous materials and understanding it is key to using amorphous materials in medicines. But what is it? And what happens to a material during it? Well, that’s what we’re going to discuss! So if that sounds good, get yourself a drink, I as ever have coffee, and let’s make a start.
Amorphous means ‘lack of form’ and in the context of chemistry an amorphous material is one where the molecules it contains are not aligned in a repeating pattern – this is in contrast to a crystalline material, where the molecules are very much arranged in specific, repeating patterns.
A material being amorphous has a number of consequences. For one, because there is no pattern of molecules it’s very hard to define an amorphous material’s structure. Random is random! But is it possible for there to be different degrees of randomness? Yes, I think it is and while you may think that’s just a theoretical argument with no practical consequence, I can tell you that the molecules in an amorphous material will in fact change their position over time – this means that the structure of amorphous materials is constantly shifting, changing their physicochemical properties, and yet because they are always random we can’t define these structures! That leads to a lot of confusion about how amorphous materials behave, as we will look at in another video.
But there is another thing that amorphous materials do which is very important to characterise, if we are to use them in medicines, yet is quite misunderstood – they exhibit a glass transition. Glass transitions are tricky to understand and easy to misinterpret, but if you can understand what happens to an amorphous material as it moves through its glass transition then I think you’ll be very well set to understand all the other funny things amorphous materials do.
First off then, what exactly is a glass transition? Would it surprise you to know it’s the temperature at which an amorphous material forms a glass? Helpful? No, not really. The term glass transition comes from, funnily enough, the glass making industry. I don’t know if you’ve ever wondered what glass is made from, but I can tell you the principal component is sand, as well as some other materials like soda ash and limestone. These materials are very clearly opaque solids at room temperature so how do they form a transparent solid?
The answer is that they are mixed together and heated up so they melt – at this point the molecules are free to move around because they are in the liquid state. Then the mixture is cooled down, and it’s this cooling step that’s critical in making the glass. When a material is crystalline all its component molecules are arranged in a perfect, repeating, ordered pattern. When the melting temperature is reached the molecules transition from this ordered state to a liquid, disordered state. Being randomly ordered means there is no need for the molecules to arrange in a pattern – they can be wherever they like! This means that the process of moving from ordered to disordered happens very quickly.
The situation is very different, however, when a material is cooled down and transitions from a liquid state to a solid state. Now the molecules must move from being randomly distributed to being perfectly aligned and as I think you might imagine this takes some time because there are trillions and trillions of molecules in even the smallest sample. If you’ve ever tried to grow crystals of a material you’ll know it can take a very long time (days or weeks in some cases) and this is why.
So when we heat a material to melt it the molecules transition from ordered to disordered and this happens instantly, while when we cool a material the molecules transition from disordered to ordered and this takes a lot of time and it is the fact it takes time that results in the creation of an amorphous phase.
Let’s look at that in more detail with the help of a diagram.
On the x-axis I have temperature and on the y-axis I have energy or volume, because it helps to have both terms to explain amorphous materials as we’ll see in a bit.
The blue line shows what happens when we heat or cool a crystalline material. Starting here at the bottom left our material is a solid. As we raise the temperature we give the material more energy and its volume expands. When we give the material so much energy that the bonds holding the molecules together in the crystal lattice can be broken the material melts and there is a sudden jump in energy or volume as the material becomes a liquid. This happens at the melting temperature of course. As we carry on raising the temperature the energy and volume of our liquid also increase.
We can of course cool the liquid back down and as we do that the energy and volume decrease. When we get to the melting temperature now the molecules should condense and crystallise, with a concomitant decrease in energy and volume. In principle then we should be able to heat and cool a crystalline material and follow this line up and down.
In practice, however, we must remember what I said just now, that when we heat a material to melt it the molecules transition from ordered to disordered, and this happens instantly, but when we cool a material the molecules transition from disordered to ordered and this takes time. What does this mean from the perspective of this diagram? Well, if we start with a crystalline solid and heat it, it will melt and form a liquid. So far so good.
But what happens on cooling? Initially we follow this line, reducing energy and volume as the temperature decreases. When we reach the melting temperature the molecules should condense and crystallise. BUT, as we’ve already discussed, this requires each and every molecule to arrange with its neighbours in a perfectly aligned crystal structure and this takes time. Obviously if that time is a few seconds then we will follow this line and form a crystal. But if that time is a few minutes, or hours, or days then what will happen? Well, if we held the sample at the melting temperature it will eventually crystallise – this is termed annealing. But imagine we don’t do that and we continue cooling. What will happen is that the molecules won’t have time to form a crystal and so the sample will remain a liquid and will follow this line instead. We have a material that is a liquid at a temperature below the melting temperature of its crystalline form – we call that a supercooled liquid.
As you might imagine, a supercooled liquid is thermodynamically unstable because really the molecules want to crystallise. The only thing they need to do that is time, because crystallisation really just involves movement of molecules. All the molecules need to move into perfect alignment. But as the temperature gets colder and colder the molecules have less and less energy and so move ever more slowly. In other words, as the temperature reduces, the time needed for crystallisation to occur gets longer and longer, so the material is essentially trapped as a supercooled liquid.
Now, you might have noticed something potentially troubling here. If we keep reducing the temperature, such that we extend this line, there will become a point at which the energy or volume of the supercooled liquid will be lower than that of the crystalline solid! That is a problem, because it suggests that a randomly arranged collection of molecules will occupy a smaller volume that the perfectly arranged crystalline solid. This apparent paradox has a name; the Kauzmann paradox and the temperature at which it would occur is called the Kauzmann temperature, Tk.
Given that our diagram appears to break the laws of physics then, and I personally won’t allow the laws of physics to be broken in my lab because the paperwork associated with that is horrendous, something must happen to ensure the lines do not cross. And what happens in reality is that there will be a break, or discontinuity, in this line, like this. This ensures the lines never cross and the energy or volume of the supercooled liquid is always higher than that of a crystalline form. Phew!
The temperature at which we see the discontinuity is called the glass transition temperature and it is the point at which the supercooled liquid forms a glass.
Cool. Literally. But what has actually happened to our material to cause it to form a glass? Now that is harder to explain and there is not actually one clear theory in the literature that I can point you too, but what I can do is say that I have read lots of the theories and have my own view of what is happening, which I am happy to share with you.
I think that glass formation results from an increase in viscosity of the material. If you think about our supercooled liquid at this point, above the glass transition temperature, then it is like a liquid – the molecules are randomly arranged and can move reasonably fast. Not fast enough to crystallise mind you, but fast enough that if we were to change the temperature slightly, higher or lower, we would see a concomitant change in the movement of the molecules. In this region therefore, the material is said to be in structural equilibrium. That is, as we change temperature we see a change in molecular mobility and the material can be thought of as a low viscosity liquid. As we cool the material down, the molecules have less and less energy and so move about less quickly. This causes the volume of the material to reduce. But importantly, the number of molecules remains the same. So as the volume reduces we have more and more molecules in a given volume. This will have the effect of increasing the viscosity, because more molecules in the same space will mean they bump off each other more frequently. So as we reduce temperature and the material follows this line the viscosity is getting higher and higher.
At the glass transition temperature the material reaches a critical point where the viscosity becomes so high it becomes a solid. That is, if we were physically to hold the material at this point it would feel solid. And that’s all a glass is; a high viscosity liquid. It looks like a solid to us, but we must remember that the molecules are randomly arranged so it is in fact a liquid, just a liquid that moves very, very slowly. So slowly in fact that it appears to be a solid. At this point the material is said to be out of structural equilibrium.
So my view of moving along this line with temperature is that the material is always a liquid, but its viscosity is always changing; below the glass transition temperature the viscosity is so high the material appears solid, and above the glass transition temperature the viscosity is lower so the molecules can move about.
Now, if you want to see what this looks like for real, here is a video I made of indomethacin being heated and cooled. Initially we can see indomethacin is a white, crystalline powder. Its melting point is around one hundred and sixty degrees Centigrade, so when the temperature increases above this we see it melt. We also see it turn yellow, which in this case does not mean the drug is degrading. For some reason indomethacin is yellow as a liquid, but that’s why I chose it for this example! When we cool indomethacin back down, and I’ve speeded the video up for this as it took some time, we see indomethacin remains as a yellow liquid. It doesn’t crystallise because the molecules don’t have time to do so. Instead, it forms a glass.
Being a glass the sample should appear solid, and indeed I can lift it off the foil with tweezers. So there’s no doubt it’s a solid. But it’s also yellow, which as I’ve already told you means it’s a liquid! So it’s a high viscosity liquid. I know that’s a tricky concept, but I hope the example helps you visualise it.
So that’s what a glass transition is – the point at which the viscosity of a supercooled liquid becomes so high it looks and feels like a solid.
Now, knowing what the glass transition temperature of a material is, is very important. I think you might imagine that an amorphous material stored below its glass transition temperature should be really quite stable but that stability will reduce a lot at temperatures above the glass transition temperature, simply because the molecules will be able to move about more, and moving is what leads to crystallisation. The glass transition temperature of indomethacin, if you’re wondering, is around forty two degrees Centigrade, but we’ll look at the consequences of that in another video.
For now though, if you remember that an amorphous material is really nothing more than a high viscosity liquid you should be good to go.
So there we are. Amorphous materials form because they can’t or won’t crystallise, usually because they have been cooled too quickly. As they cool down they form a supercooled liquid. The colder the supercooled liquid becomes, the more viscous it gets. The glass transition temperature is the point at which the viscosity of the supercooled liquid becomes so high the material looks and feels like a solid, and at this point it’s said to be a glass. And on heating an amorphous glass won’t melt – it will go back to being a low viscosity liquid.
I hope you found that useful. If you did please hit the like button, subscribe if you haven’t already and please tell your friends about the channel – all of that really helps. And if there are topics you’d like me to discuss, please leave a comment below. Otherwise, thank you so much for watching and I’ll see you again soon.
Source: Simon Gaisford, Unlocking the Mystery of Glass Transition Temperatures – YouTube
Read also others Simon Gaisford articles here:
- The science of pharmaceutical suspensions explained!
- What is BCS and why it’s quite useful?
- Partitioning & Partition Coefficient: Definition and explanation by Pharma Drama
- Probiotics for constipation and gut health in children and young people with Down’s syndrome
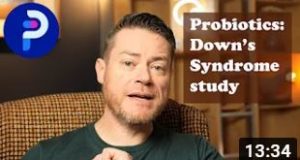