An insight on lipid nanoparticles for therapeutic proteins delivery
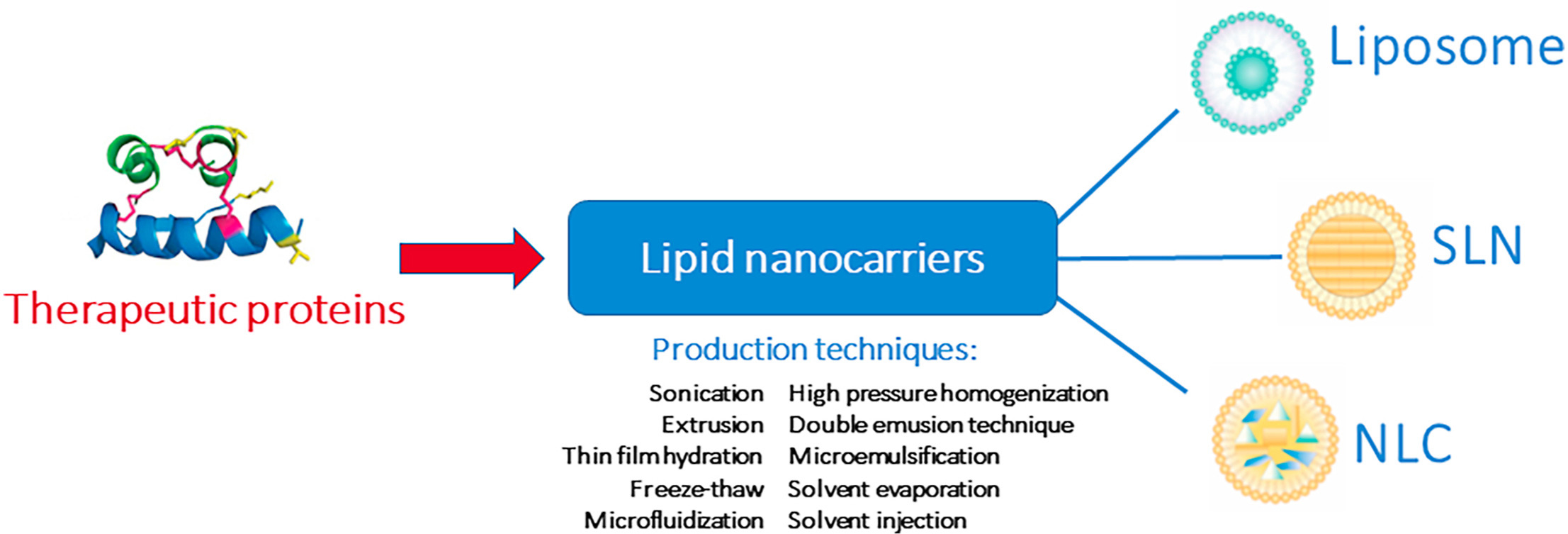
Therapeutic proteins are well-tolerated bioactive compounds used in different therapies, due to its high specificity and biopotency. Nevertheless, they may also present some physicochemical instability, leading to loss of bioactivity hampering treatments. This can be avoided by its loading into lipid nanoparticles, which are biocompatible and biodegradable carriers. The use of lipids nanoparticles to deliver therapeutic proteins overcomes different challenges, allowing its administration by all delivery routes.
Highlights
- The delivery of therapeutic proteins to the body is challenging.
- Lipid nanoparticles overcome the challenges of therapeutic proteins delivery.
- Different methods are used to tune protein-loaded lipid nanoparticles features.
- The maintenance of proteins structure and bioactivity upon encapsulation is crucial.
- Further research is needed on developing NLC for therapeutic proteins delivery.
Thus, therapeutic proteins may be loaded into liposomes, the first developed lipid-based nanocarriers composed of phospholipid bilayers, solid lipid nanoparticles composed of a solid lipid matrix, or nanostructured lipid carriers made of a blend of liquid and solid lipid as matrix. The latter are currently marking the trend in lipid nanocarriers due to its high loading capacity, good stability upon storage and better sustained release pattern. Production methods must focus both on attaining the desired nanocarrier features, and maintenance of therapeutic proteins structure and bioactivity. This review aims to make an insight overview on the application of lipid nanoparticles to deliver therapeutic proteins, showing its potential in different therapies. A special focus is given to the production techniques to obtain therapeutic proteins-loaded lipid nanoparticles.
Chapter 5.2 Solid lipid nanoparticles
SLN were developed by Schwarz et al. (1994) and in parallel by Morel et al. (1998) [79,80]. SLN brought attention due to its advantages, being able to assemble the advantages of other colloidal carriers while avoiding some of their disadvantages. These nanoparticles are interesting delivery systems that have shown great advantages including:
Allowing controlled drug release and targeting
-
Increasing drug stability
-
Allowing high drug payload
-
Incorporating lipophilic and hydrophilic drugs
-
Being composed of biocompatible lipids
-
Large-scale production ability
-
Use of Generally Recognized as Safe (GRAS) compounds and therefore low toxicity as carriers
-
Improvement of drug stability and safety
They have been actively investigated for the delivery of drugs by different delivery routes [50,81,82]. The most frequently used excipients for SLN production are shown in Table 5.
Table 5. Excipients for SLN and NLC production. Adapted from Ref. [76].
Excipients | Examples | Properties |
---|---|---|
Solid lipids | Beeswax | Natural wax with GRAS status and MP of 62–64 °C; requires HLB of 9 |
Carnauba | Natural wax with GRAS status, MP of 82–85 °C; requires HLB of 12 | |
Cetyl palmitate | Synthetic wax with MP of 40.5–51 °C; requires HLB of 10 | |
Compritol® 888 ATO | Blend of esters of behenic acid with glycerol; MP of 69–74 °C Acceptable safety profile and established as emulsifier, with HLB of ≈2 |
|
Dynasan® | Series of natural and safe triglycerides with different MPs | |
Gelucire® | Series of lipid defined by their MP between 33-70 °C and HLB between 1-18 Gelurice 50/13 is GRAS listed and the most frequently used for SLN/NLC |
|
Precirol® ATO 5 | Glyceryl palmitostearate, mixture of mono, di and triglycerides of palmitic and stearic acid, with GRAS status, MP of 58 °C and HLB of 2 | |
Softisan® 378 | Blend of triglycerides with hydrocarbon with GRAS status and MP of 35–42 °C | |
Stearic acid | Endogenous fatty acid with GRAS status, MP of 70 °C and HLB of ≈15 | |
Liquid lipids | Miglyol® 812 | Triglycerides of capric and caprylic acid with GRAS status, high stability against oxidation and high solubility for several drugs |
Oleic acid | Pure substance used as emulsifying agent and penetration enhancer with GRAS status | |
Squalene | Triterpene produced by human skin cells (as precursor for cholesterol) | |
Vitamin E/alfa-tocopherol | Offers sensitive substances protection against oxidation | |
Surfactants | Lecithin | Component of cell membranes, used in a wide variety of pharmaceutical applications as emollient, emulsifying and solubilizing agent, with HLB between 4–9 |
Plantacare® 810 | Caprylyl/capryl glucoside, high effective stabilizer for SLN and NLC with HLB of 15–16 | |
Poloxamer® 188 | Used as emulsifier and stabilizing agent in a wide variety of pharmaceutical formulations, it is nontoxic and non-irritant, with HLB >24. | |
Quillaja saponin | Natural saponin-based surfactant with antioxidant properties and HLB of 13.5 | |
Sodium lauryl sulphate | Anionic surfactant, widely used in cosmetics and pharmaceutical formulations, moderately toxic but with GRAS status and HLB ≈40 | |
Tween® 80 | Polysorbate 80, an O/W surfactant with GRAS status widely used and HLB of ≈15 |
The lipids compose the matrix of the SLN, and are solid at room and body temperature, usually with a melting point above 40 °C, used in a concentration ranging from 5 to 40%. Distinct types of lipids are used, ranging from triglycerides, partial glycerides, and fatty acids to steroids and waxes. This fact is one of the major advantages of SLNs, as they are made of physiologic materials decreasing the danger of acute and chronic toxicity of these nanoparticles. The choice of lipids relies on the solubility of the compound that is incorporated inside the matrix [63,83].
The emulsifier role in the formulation is to reduce the surface tension between the aqueous and lipid phases, thereby helping the stabilization of the system. Since they are amphiphilic molecules, they are placed in the interface of the system [50,81]. Several types of emulsifiers have been employed in SLN formulations, like as bile salts, ethoxylated alcohols, fatty acids, phospholipids, poloxamers, polyethylene glycols, polysorbates, polyvinyl alcohols, quaternary ammonium compounds, sorbitan esters, and tyloxapol, and it was discovered that a binary combination of emulsifiers helps to stabilize the systems more effectively and results in smaller nanoparticle sizes [63]. The choice of emulsifiers should take into consideration the hydrophilic-lipophilic balance (HLB) of the lipids employed in the formulation, as well as their concentration of the lipid phase and the administration route [50,81].
There are three incorporation models for the SLN that differ in the location and distribution of the loaded therapeutic protein within the lipid core as shown in Fig. 8.
-
SLN Type I/homogeneous matrix model – In this model the drug is dispersed in the lipid core or as amorphous agglomerates. This type is usually produced by high pressure homogenization (HPH), either cold HPH or hot HPH with an optimized drug/lipid ratio. Usually, these nanoparticles show good controlled release properties.
-
SLN Type II/drug enriched shell model – In this model it is obtained a drug-free lipid core surrounded by an outer shell containing the drug and the lipid. This model is used when a faster release of the encapsulated drug is desired.
- SLN Type III/drug enriched core model – In this model, the core of the nanoparticle is enriched with drug while the lipid is in the outer shell. This morphology is obtained when the drug concentration in the melted lipid mass is close to its saturation solubility and the lipid, when cooled, precipitates in the core before the lipid. This model is also suitable for drugs that require a prolonged drug release [68,84].
![Fig. 8.Types of SLN. Reprinted from Ref. [78].](https://ars.els-cdn.com/content/image/1-s2.0-S177322472200750X-gr8.jpg)
Nevertheless, the SLN has two main limitations related to its densely packed crystal structure: low loading capacity and drug expulsion during storage. Both lipophilic and hydrophilic active substances can be entrapped [63]. Drugs are mostly incorporated between the fatty acid chains, lipid layers, or in the amorphous clusters of the crystal imperfections. SLN usually crystallizes in a perfect lattice, especially those obtained by highly purified lipid, which explains the low encapsulation efficiency, since the more densely packed the crystal is the less drug is possible to incorporate [85]. Furthermore, during storage, the lipid molecules suffer a time-dependent restructuration process in which the more perfect lipid crystalline structures lead to the expulsion of the drug [86]. Additionally, SLN dispersion may suffer gelation phenomenon once its viscosity increases during the cooling process which results in a viscous gel and consequently leads to an increase in particle size and particle agglomeration [63].
In a study where bovine serum albumin (BSA) was used as a model protein for the encapsulation into a matrix modified by incorporation of lecithin into the lipid matrix and different emulsifier concentrations, the obtained particle payload with BSA was between 2.5 and 15% and seemed to be commanded by the particle surface characteristics, particularly the surface charge and the specific surface area [87]. In a different study, using SLN for the encapsulation of lysozyme, the method used produced formulations with reduced concentration of protein and low encapsulation efficiency, which considering the excessive costs of some therapeutic proteins and the waste generated by a reduced encapsulation efficiency, represents a limiting factor, and urges the need of developing of further improved lipid nanoparticle formulations [88]. In Table 4 it is summarized more works focusing on SLN for protein delivery.
Download the full study as PDF here An insight on lipid nanoparticles for therapeutic proteins delivery
or read it here
Cláudia Viegas, Fatumata Seck, Pedro Fonte, An insight on lipid nanoparticles for therapeutic proteins delivery, Journal of Drug Delivery Science and Technology, 2022, 103839, ISSN 1773-2247,
https://doi.org/10.1016/j.jddst.2022.103839.