Superdisintegrants and their working mechanisms- by DFE Pharma
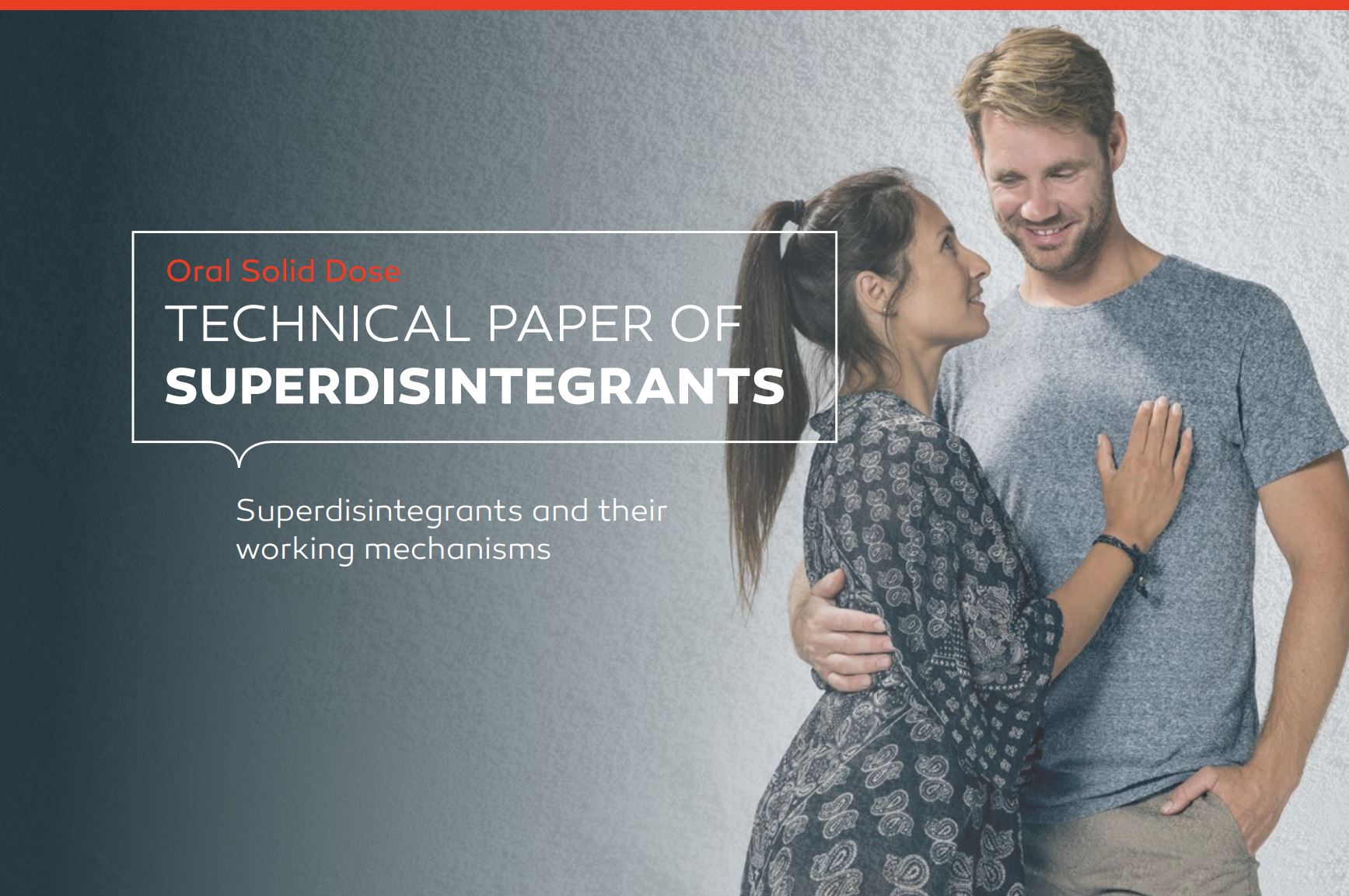
Superdisintegrants and their working mechanisms
Disintegrants and superdisintegrants are well-known excipients that are used in the formulation of oral dosage forms. They promote disintegration of tablets and capsule slugs when placed in an aqueous environment. Disintegration is the process of breaking up a dosage form in smaller particles to increase the available surface area. It is important as tablet disintegration could determine the drug availability for dissolution and subsequently absorption. There are many factors in a formulation that affect the disintegration of a dosage form. These include proportion of the disintegrant, type of tablet matrix, solubility of tablet matrix, hardness of tablet, API dose, API properties, tablet’s porosity, and the working mechanism. The main mechanisms of disintegration are swelling, wicking and deformation.
Mechanisms of action
Promotion of disintegration in a formulation can be achieved by the addition of a disintegrant or superdisintegrant. Five mechanisms of action are proposed for (super-) disintegrants: heat of wetting, particle repulsion, wicking/capillary action, swelling and deformation recovery (Carmella et al., 1988, Bele & Derle, 2012). It is believed that no single mechanism is responsible for the action of disintegrants, but that disintegration is the results of inter-relationships between these mechanisms. The most widely accepted mechanisms are swelling, wicking and deformation recovery (Joshi et al., 2015).
Swelling
The most accepted working mechanism for tablet disintegration is by swelling. Swelling is the result of absorbed solution. It is associated with multi-dimensional amplification where particles enlarge in all directions to create an internal pressure. This pressure pushes apart adjoining components and the matrix breaks when the adhesiveness of ingredients is overcome (Desai, Liew & Heng, 2016).
For swelling disintegrants, porosity of tablets could have a big impact on disintegration performance. A porous tablet matrix with large void spaces could reduce the impact of swelling and impede their efficiency in tablet disintegration. On the other hand, low porosity compacts could hinder liquid entry and prolong disintegration as well (Desai, Liew & Heng, 2016).
Wicking (capillary action)
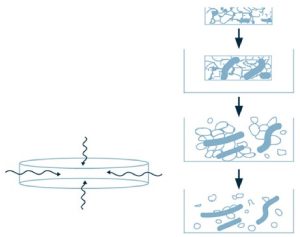
Wicking may be defined as the phenomenon of drawing water into the tablet due to the presence of hydrophilic groups. It is the capillary action by which water penetrates a tablet through the porous structure of the disintegrant. Disintegrants with low cohesiveness and low compressibility provide tablet porosity with pathways for the penetration of fluid into the tablets (Mohanachandran, Sindhumol, & Kiran, 2011). As water is pulled into the structure, the physical bonds between the dosage components is reduced and the matrix falls apart, as indicated in Figure 1.
Deformation / strain recovery
Strain recovery is the reversible process of deformation. It is the result of plastic deformation of disintegrants that are believed to be elastic in nature. During compaction, the particles of these disintegrants deform and interparticle bonds are formed. When these compacted disintegrants come in contact with the aqueous media however, mechanical activation helps to partially recover their original shapes (Desai, Liew & Heng, 2016). By returning to the original state, a pressure within the tablet matrix is obtained, resulting in disintegration (Shihora & Panda, 2011).
Solani amylum is an example of a disintegrant with deformation mechanism. It is a native potato starch and can be used in a concentration of 3-15%w/w in a tablet formulation as a disintegrant. Solani amylum is more effective when used with high compaction pressures, because of the higher deformation for the potato starch particles, making the strain recovery stronger (Szabó-Révész & Szepes, 2009).
Superdisintegrants used in dosage forms
Disintegrants are commonly classified in literature as traditional disintegrants and superdisintegrants (Moreton, 2008). Traditional disintegrants include native starches, alginic acid, ion exchange resins and microcrystalline cellulose.
The term superdisintegrants was introduced shortly after sodium starch glycolate, croscarmellose sodium and crospovidone became available. Superdisintegrants have improved efficiency and facilitate faster disintegration with smaller quantity, compared to regular disintegrants. Commonly used superdisintegrants are highly efficient at low concentration levels (2-5%w/w).
Sodium starch glycolate – Primojel®
The first introduced superdisintegrant in the market was sodium starch glycolate (SSG). Figure 2 shows a SEM picture of Primojel®, DFE Pharma’s sodium starch glycolate. It is produced via a two-step reaction, starting with a potato starch structure.
The first reaction step involves introduction of cross links across the amylose and amylopectin chains. The function of the cross-links is to reduce the water-soluble fraction and the viscosity of the substituted starch in water. Crosslinks in SSG provide high spacing between the chains, facilitating water penetration and swelling. A second step is carboxymethylation, which involves the substitution of hydroxyl groups by sodium carboxymethyl ester groups. This substitution disrupts hydrogen bonding within the polymer structure, resulting in the ability to take up large amounts of water without the formation of a gel.
This unique structure of SSG makes that compared to other types of superdisintegrants, SSG has the highest swelling value (see Figure 3). Primojel® can take up more than 20 time its own weight of water and it swells in three dimensions. Rapid water penetration into the tablets ad powerful swelling results in rapid disintegration.
The swelling action makes Primojel® most effective in non-soluble matrices. With soluble or partially soluble matrix components, the matrix is dissolving and thus the disintegrant is deprived of some of what it might push against, thus reducing the disintegrant effect (Moreton, 2008).
Croscarmellose sodium – Primellose®
Croscarmellose sodium is a cross-linked polymer of carboxymethyl cellulose. It is manufactured from high quality wood pulp or cotton liners. Figure 4 shows a SEM picture of Primellose®, which originates from cotton liner.

The production of Primellose® starts with carboxymethylation of cellulose chains. This step reduces the hydrogen bonds in the cellulose chains, resulting in a porous structure. A second step is cross-linking, which in this case is not performed by an external agent, but via some of the carboxymethyl groups themselves. The degree of cross-linking is related to two properties of the disintegrant, namely the water-soluble content and the settling volume. Both these parameters reduce as the degree of cross-linking increases.
Croscarmellose sodium appears to act partly through swelling, but it only swells in two directions (Mohanachandran, Sindhumol & Kiran, 2011). The main working mechanism of CCS is wicking, evidenced by the fibrous particle shape. The long fibres will function over a longer distance in the tablet matrix and thereby cause disruption over a longer distance than crospovidone or SSG particles (Morton, 2018).
Figure 5 shows the disintegration time of placebo lactose tablets with different concentrations of SSG (Primojel®) and CCS (Primellose®). In soluble matrices, CCS often is most effective in getting the lowest disintegration times. The figure also shows the impact of filler-binder on the disintegration of a tablet. When disintegration of the tablet is critical, SuperTab® 30GR is the preferred type of lactose as s filler-binder.

Crospovidone
Crospovidone is a cross-linked polyvinylpolypyrrolidone. This polymer is also known as a ‘popcorn’ polymer, as it possesses a popped structure like indicated in Figure 6.

The production of crospovidone is performed by proliferous polymerization of vinylpyrrolidone (Barabas & Adeyeye, 1996). This process takes place via a free radical, which can be developed through rupture of some polymer chains or it can be introduced via a free radical initiator. The growth of chains can be initiated at multiple positions, and is therefore not limited to one position, which often results in high degrees of cross-linking.
No clear consensus on the working mechanism of crospovidone exists. Wicking followed by secondary swelling was proposed by some first (Kornblum & Stoopak, 1973), while others propose high swelling pressure as the disintegration mechanism (Shu et al., 2002). Strain recovery is also mentioned in literature as the main disintegration mechanism of crospovidone (Quodbach et al., 2014). Although unclarity exists on the exact working mechanism, crospovidone is generally considered as a very effective superdisintegrant. A potential drawback of crospovidone however, is that it can contain traces of formaldehyde and peroxides which may have implications for compatibility with other components in the formulation (Morton, 2008).
See the full technical paper on Superdisintegrants by DFE here
(click the pictures to download the technical paper)
Source: DFE Pharma, technical paper “Superdisintegrants“