Flow behavior under shear cell analysis of two HMPC grades
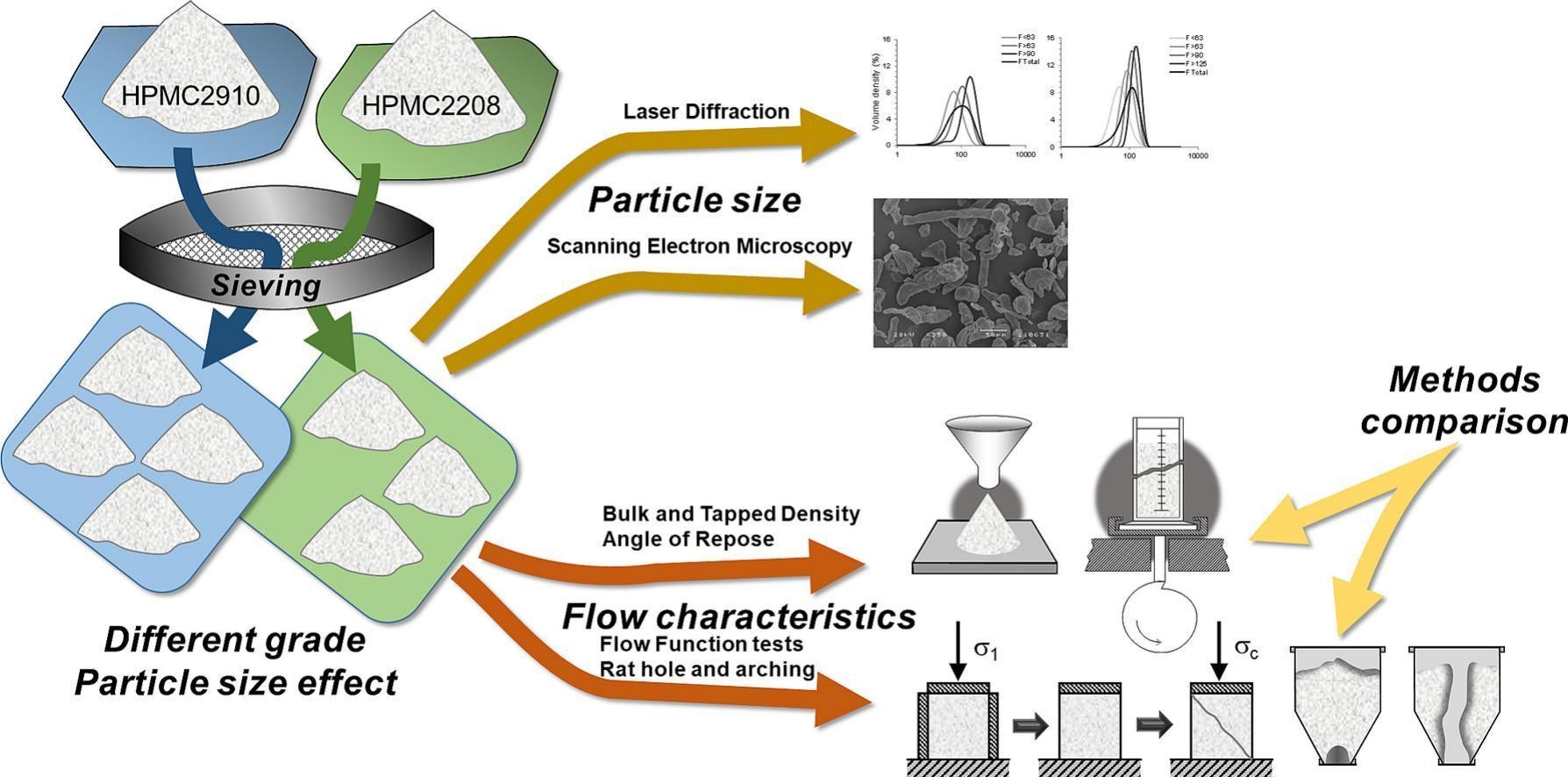
Abstract
A comparative analysis was conducted to examine the flow characteristics of two HPMC grades and their fractions. Excipients processing contains critical steps in the manufacturing of solid dosage forms and therefore it is essential to determine their flowability. Flow characterization was done using conventional and shear cell methods. After the division into different particles size, HPMC2910 showed an increase of the ffc for the fractions with the largest particle size and HPMC2208 showed an unprecedented result for the fraction with the smallest size. Thus, for this excipient, the best ffc was obtained with the fraction containing the smallest particles which surprisingly contradicted the literature (shape effect overlapped the size effect). It was also found for different fractions that the ffc increased with the increasing of the σ1, showing in some of them a decrease of the same from a certain σ1 value. Furthermore, caking was not observed in both cases.
Introduction
The effectiveness of solid oral dosage forms (SODFs) deeply depends on the properties of their excipients. Incorrect inclusion can cause compressing problems, difficulties with capsules, sachets, batch inconsistencies, and other variations in the final products. The amount of excipients in a formulation can reach up to around 90% of its mass and, therefore, its compressibility properties play a fundamental role in the manufacturing process [1,2]. The excipients used in SODFs can be classified into those that affect the compressibility characteristics (diluents, binders, lubricants, glidants and anti-adherents) and those that affect the biopharmaceutical behavior and the physical-chemical properties (disintegrants, flavorings, sweeteners and colorants) [3]. They can also be divided into modified, co-processed and novel excipients [4]. In addition, the terms “multifunctional excipients” and “high functionality excipients” also appear [5].
One of the most used excipients is hydroxypropyl methylcellulose (HPMC), which is mainly used as a binder for tablet manufacturing [6], as a matrix for prolonged release [7,8] and for film-coating [9,10]. The HPMC amounts used may vary according to the intended function: 2 to 5% (w/w) as a binder and 10 to 80% (w/w) for prolonged release of drugs from hydrophilic matrices [11]. The degree of substitution and proportion of methoxy/hydroxypropyl groups markedly influence their physical-chemical properties such as organic solubility [12], swelling, powder flow, compressibility, drug diffusion and release from pharmaceutical systems [13]. According to the European Pharmacopoeia (11th Edition), various types of this polymer are distinguished by the 4-digit code given behind the non-trade name (HPMC 1828, HPMC 2208, HPMC 2906 and HPMC 2910) [14]. The first two digits (HPMC 2208) indicate the average percent of methoxy-groups (-OCH3), while the last two digits (HPMC 2208) designate the average percent of hydroxypropoxy-groups (OCH2CH(OH)CH3), as calculated on a dry sample weight basis [13,15]. The Dow Chemical Company divides Methocel® [16] with respect to its degree of substitution into subtypes K (HPMC 2208), E (HPMC 2910), F (HPMC 2906) and J (HPMC 1828) and adds a suffix that indicates the viscosity of the solution corresponding to the molecular weight of each polymer. The number that follows is obtained by measuring the viscosity (mPa.s) in a 2% (w/w) aqueous solution at 20 °C and are designated by “C” (multiplier of 100) or “M” (multiplier of 1000). Also, the last suffix is used to identify the grade of the product, such as premium (P), low viscosity (LV), controlled release (CR), granular (G), direct compression (DC), surface treated (S) or food grade (FG) [17]. The HPMC viscosity range is 3–100,000 mPa.s [18].Flow properties depend on forces between particles, such as van der Waals, liquid bridges, electrostatic, capillary, and mechanical interlocking. They result from interactions between them [[19], [20], [21], [22]], between them and the equipment surfaces [[19], [20], [21], [22], [23]], and dependent on the environmental conditions and other factors (static electricity and gravity force) [24].
To determine the flow properties there are several methods described in Ph. Eur., such as Conventional methods (Conv methods), that include the Angle of Repose, Compressibility Index (CI) and Hausner Ratio (HR), and Shear Cell methods, which allows to determine the Flow Index (ffc) [19,[25], [26], [27], [28]]. The ffc allows to numerically characterize the flowability relating the unconfined yield strength (σc) with the major principal consolidation stress (σ1). The curves σc vs σ1 obtained are derived from the Mohr-Coulomb method and are designated as an instantaneous flow function (IFF) for storage period (t) = 0, or as time flow function (TFF) when the storage period (t) > 0 [19,22,[29], [30], [31]]. The flow function (FF) can be expressed only by cohesion and pre-consolidation stresses, without the interference of any friction coefficients [32,33]. In addition, Shear Cell methods also allow the determination of other parameters, such as CI, HR, effective angle of internal friction (φe), effective angle of wall friction (φx), critical arching and critical ratholing. Knowledge of φe and φx makes it possible to predict the inclinations of the wall surfaces of the equipment and the dimensions of the outlet [21,31,34,35]. According to Jenike, the arch formation can only occur when the ffc is below 1.3 (very cohesive powder) [36]. During the discharge processing, the external stress (σ0) is mainly dependent on the powder density as well as the outlet diameter and weakly dependent of the σ1. For a higher σ1 is usually observed a higher σc, and therefore is more likely σc to exceed the σ0. When this happens, the possibility of flow obstruction is greater. The ratio σ1 vs σ0 is designated as the factor flow and σ0 has been described by Jenike as the stress “at the abutment of the dome” [37,38]. Thus, the outlet flow is dependent on two contradicting factors (σ0 and σc) [19,37,39].
In this work, as in others already carried out by the same authors, the main focus of the research has been trying to understand how the shape and size effect of the particles affect the flow of bulk powders and their fractions obtained by sieving. The variability of size and shape is very wide, which may give rise to fractions of small size and constant or varied shape or fractions with a larger range of size with constant or varied shapes. The interaction between these two factors can be additive or contradictory, leading to the most varied flows possible. However, the predominance of one factor over another may contradict what is defined in the literature, which is that as the size decreases the powder flow worsens. The novelty of this work in addition to other surprising results was precisely one of the excipients to present the fraction with smaller particle size with the best flow demonstrating the predominance of shape over size. In addition it was possible to select the best fraction of each excipient for its use in the formulation of new SODFs that can be any of them including the smaller particle size as well as the bulk fraction.
Read more here
Materials
HPMC2208 (METHOCEL® K4M Premium, batch – D180KA1002, DuPont_USA) and HPMC2910 (VIVAPHARM® HPMC E15, batch – 81,015,201,815, JRS PHARMA, Germany)
Paulo J. Salústio, Beatriz V. Pais, Teresa Malta, Telmo Nunes, José P. Sousa e Silva, Paulo J. Costa, Flow behavior under shear cell analysis of two HMPC grades, Powder Technology, 2023, 119025, ISSN 0032-5910, https://doi.org/10.1016/j.powtec.2023.119025.
See the webinar:
“Enhanced Stability and Bioavailability of Poorly Soluble APIs“,
18 October 2023:
Get more information & register here for free:
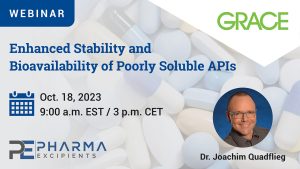