Predicting pharmaceutical inkjet printing outcomes using machine learning

Inkjet printing has been extensively explored in recent years to produce personalised medicines due to its low cost and versatility. Pharmaceutical applications have ranged from orodispersible films to complex polydrug implants. However, the multi-factorial nature of the inkjet printing process makes formulation (e.g., composition, surface tension, and viscosity) and printing parameter optimization (e.g., nozzle diameter, peak voltage, and drop spacing) an empirical and time-consuming endeavour. Instead, given the wealth of publicly available data on pharmaceutical inkjet printing, there is potential for a predictive model for inkjet printing outcomes to be developed. In this study, machine learning (ML) models (random forest, multilayer perceptron, and support vector machine) to predict printability and drug dose were developed using a dataset of 687 formulations, consolidated from in-house and literature-mined data on inkjet-printed formulations. The optimized ML models predicted the printability of formulations with an accuracy of 97.22%, and predicted the quality of the prints with an accuracy of 97.14%. This study demonstrates that ML models can feasibly provide predictive insights to inkjet printing outcomes prior to formulation preparation, affording resource- and time-savings.
Introduction
Inkjet printing is a manufacturing technology based on material jetting, wherein droplets of ink are deposited onto a substrate. Inkjet printing has garnered considerable attention amongst pharmaceutical scientist for its versatility in producing personalised medicines and unique dosage forms (Alomari et al., 2015; Scoutaris et al., 2016a). Notably, inkjet printing has been used to load drugs onto orodispersible films (Alomari et al., 2018; Arshad et al., 2020; Jachowicz, 2017; Kiefer et al., 2021; Vuddanda et al., 2018), bioadhesive films for cervical administration (Varan et al., 2017), transdermal microneedles (Boehm et al., 2014; Uddin et al., 2015), coronary metal stents (Scoutaris et al., 2016b), contact lenses (Pollard et al., 2023; Tetyczka et al., 2022), and even nails (Pollard et al., 2022). Inkjet printing has also been used to dispense drug-loaded micro- and nanoparticles dispersed in the ink liquid (Akagi et al., 2014; Boehm et al., 2013; Chou et al., 2021; Lee et al., 2012; Yeo et al., 2004). Inkjet printing may also be combined with other additive manufacturing technologies to impart special features that would otherwise be unattainable with conventional manufacturing technologies. For instance, inkjet printing was used in conjunction with fused deposition modelling (FDM™) 3D printing to produce drug-loaded tablets with quick response (QR) codes printed on them (Trenfield et al., 2019). These QR codes were designed to encode patient-related information that could be read using a smartphone, and to serve as an anti-counterfeit strategy. A similar concept was also applied for the fabrication of orodispersible substrates and capsules with printed QR codes (Chao et al., 2021; Edinger et al., 2018; You et al., 2016). Potent drugs or drugs with low dose requirements are conventionally explored for inkjet printing due to the technology’s suitability for printing low dose drug products. However, inkjet printing has also been used to fabricate the entire 3D drug-loaded tablets (Acosta-Vélez et al., 2018; Kyobula et al., 2017; Sen et al., 2020) and implants (Ruiz-Cantu et al., 2021). The affordability, precise control of droplet deposition, and versatility of inkjet printing has supported the continued expansion of research in its pharmaceutical applications, resulting in a wealth of publicly available data on printing parameters and outcomes (Evans et al., 2021).
In inkjet printing, droplets are deposited either continuously (continuous inkjet) or with a drop-on-demand (DoD) mechanism. DoD inkjet printing can be further categorised based on the mechanism by which droplets are generated: thermal and piezoelectric inkjet printing (Daly et al., 2015; Hoath, 2016; Singh et al., 2010). In thermal inkjet printing, a thermal resistor present in the printhead heats the ink, inducing rapid vaporisation and consequently the formation of a vapour bubble that forces a droplet out of the nozzle. In piezoelectric inkjet printing, an electric current is applied to a piezoelectric material in the printhead, inducing mechanical deformation of the material which in turn exerts a pressure within the printhead, thereby ejecting a droplet. Due to the absence of heat application, piezoelectric inkjet printing has been popularly explored for the fabrication of personalised medicines loaded with thermally labile drugs (Eleftheriadis et al., 2020a; Eleftheriadis et al., 2020b). Interestingly, while it might be instinctive to assume that thermal inkjet printing is not amenable to heat-sensitive drugs, there is insufficient evidence demonstrating that thermal degradation of drug occurs during the printing process. This is because the application of heat in the thermal inkjet printing process last for only a few milliseconds (Scoutaris et al., 2016a).
Download the full article as PDF here Predicting pharmaceutical inkjet printing outcomes using machine learning
or read it here
Paola Carou-Senra, Jun Jie Ong, Brais Muñiz Castro, Iria Seoane-Viaño, Lucía Rodríguez-Pombo, Pedro Cabalar, Carmen Alvarez-Lorenzo, Abdul W. Basit, Gilberto Pérez, Alvaro Goyanes, Predicting pharmaceutical inkjet printing outcomes using machine learning, International Journal of Pharmaceutics: X, Volume 5, 2023, 100181, ISSN 2590-1567, https://doi.org/10.1016/j.ijpx.2023.100181.
Read more on Overview of Pharmaceutical 3D printing here:
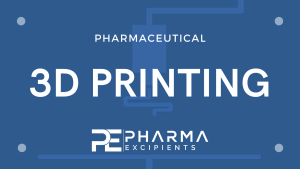