Comparative analysis of tablet dissolution behavior: Batch vs. Continuous direct compression
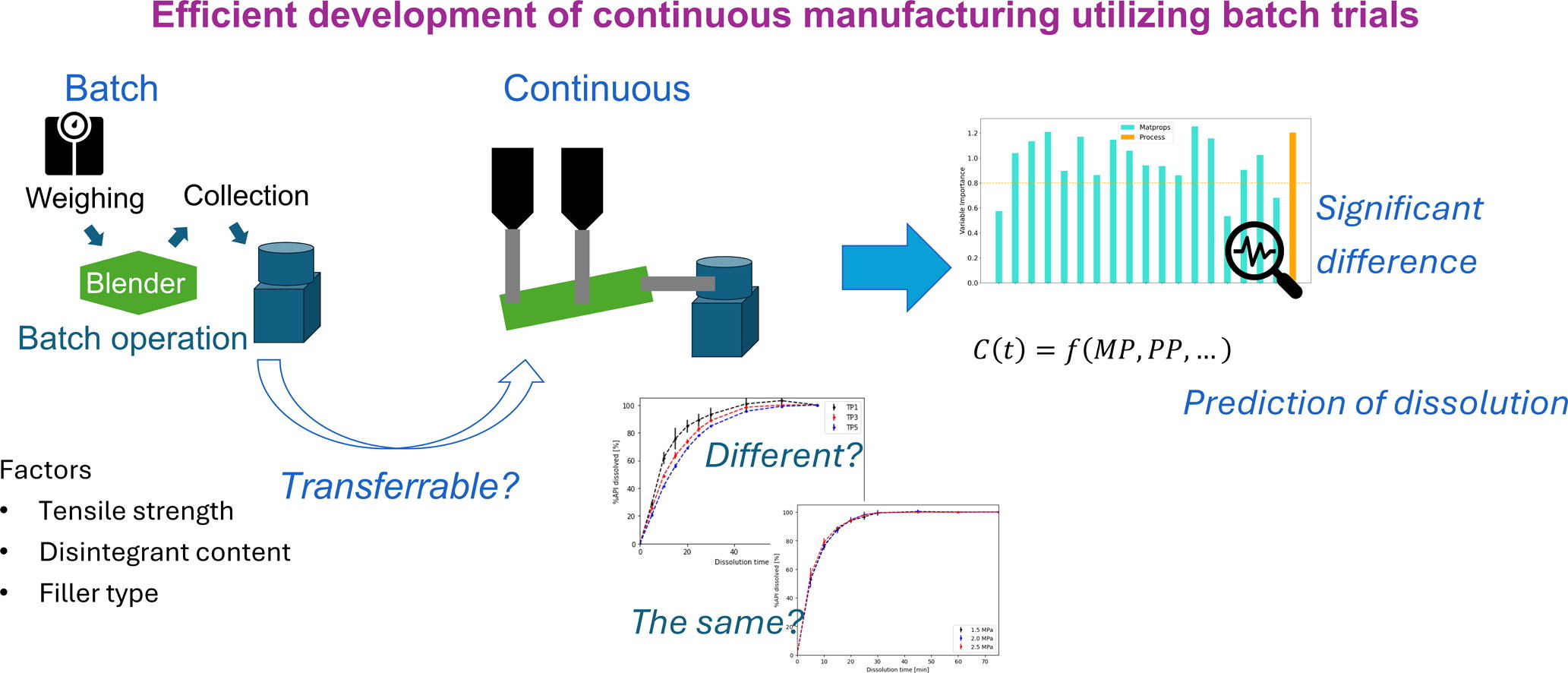
Abstract
Continuous manufacturing offers advantages over traditional batch methods, including agility, efficiency, and sustainability. However, transitioning to continuous manufacturing in process development is challenging due to the need for early adoption of industrial-scale equipment. Conversely, batch processes require extensive scale-up studies before commercialization, which continuous processes can avoid. A more efficient approach is to use batch trials in early development to design formulations and processes for continuous manufacturing, requiring assessment of their transferability.
This study compares the dissolution behavior of immediate-release tablets manufactured via batch and Continuous Direct Compression (CDC), using ibuprofen, a BCS Class II drug. A Design of Experiments (DoE) approach varied formulation properties and tensile strength, with three methods: i) similarity factor ([f2]), ii) Weibull model fitting and Partial Least Squares (PLS) regression, and iii) Gaussian Process Regression (GPR) to assess the transferability of batch trial data and dissolution models for CDC formulation and process design.
Dissolution profiles were identical between batch and CDC trials when formulations and tensile strength matched, with differences observed only due to deviations in actual tensile strength. The PLS model indicated minimal impact of operational modes on dissolution behavior. The GPR model based only on batch trial data predicted CDC dissolution profiles with a mean R2 of 0.910 and RMSE of 4.88%. Overall, the transferability analysis confirmed the predictive capacity of the developed model using batch trial data on the dissolution behavior of tablets manufactured via a CDC line.
Highlights
- Tablet dissolution was compared between batch and continuous manufacturing.
- Different mathematical models were used to analyze dissolution behavior.
- The manufacturing method had little effect on how tablets dissolved.
- A prediction model accurately estimated dissolution in continuous manufacturing.
- Batch experiments can help design continuous manufacturing processes.
Introduction
Continuous manufacturing offers several advantages over batch manufacturing. Its flexible production approach, determined by operating time rather than equipment dimensions, allows for swift adaptation to market demands, which is particularly beneficial during pandemics or drug shortages (Ierapetritou et al., 2016). Additionally, utilizing the same equipment size for both development and commercial manufacturing avoids the need for scale-up studies, saving time and valuable consumption of active pharmaceutical ingredients (APIs) during process development, (Fonteyne et al., 2015; Ierapetritou et al., 2016). Integration of all unit operations into a continuous line eliminates the need for intermediate product storage, transfer, and testing, which not only results in a shorter processing time but also minimizes errors due to human interventions, enhancing overall manufacturing reliability (Fonteyne et al., 2015, Vanhoorne and Vervaet, 2020). Moreover, continuous manufacturing requires less floor space, reduces solvent usage (when used), and minimizes waste, thus contributing to a reduced environmental footprint (De Soete et al., 2013; Lee et al., 2015; Vanhoorne and Vervaet, 2020). This enhanced manufacturing efficiency translates into more affordable pharmaceuticals or enables savings to be redirected toward the discovery of new drug products (Suresh and Basu, 2008, Vernon et al., 2007).
The adoption of continuous manufacturing would affect quality assurance, drug formulation and process development. Since final products are continuously produced during the operation, process monitoring and control become more critical for continuous manufacturing. The required quality system for continuous manufacturing matches the concept of Quality by Design (QbD), which is a systematic development approach stressing the importance of comprehensive product and process understanding under predefined objectives (ICH, 2009). Tablet dissolution is one of the critical quality attributes (CQAs) that needs to be well understood and controlled for most oral solid dosage forms. While an in-depth understanding of tablet dissolution is important in the development phase, an efficient experimental design is essential as dissolution testing can be labor-intensive.
Despite numerous merits, efficient formulation and process development are some of the most important challenges to continuous manufacturing. While many experiments can be performed using lab-scale equipment for batch trials, industrial-scale equipment is expected to be used from the development phase for continuous manufacturing (Lee et al., 2015). This would lead to less experimental work during the process scale-up, which is one of the bottlenecks in batch process development, and brings economic benefits (Matsunami et al., 2020). On the other hand, continuous manufacturing can lead to higher material amounts to run a single experiment than lab and pilot-scale batch manufacturing, e.g. due to start-up and shut-down (Matsunami et al., 2021). Consequently, the cost of continuous manufacturing would be higher than batch manufacturing in the development phase, when APIs are more expensive and limited.
One potential solution to reduce material consumption is the application of batch trials in the development phase for designing formulations and processes of continuous manufacturing. Formulations and process settings are selected based on batch experiments and transferred to continuous manufacturing. To make this possible, the obtained CQAs should be identical between batch and continuous manufacturing. So far, numerous comparative studies have been performed to understand the differences between batch and continuous manufacturing. Besides economic comparison (Matsunami et al., 2018, Schaber et al., 2011), product quality was compared between batch and continuous wet granulation methods (Järvinen et al., 2015, Koyanagi et al., 2023, Matsunami et al., 2019, Vercruysse et al., 2013).
Several differences in granule CQAs have been reported because of different mechanisms in batch and continuous wet granulators. Compared to the wet granulation method, Continuous Direct Compression (CDC), which is the simplest tablet manufacturing process, has a lot of similarities with batch direct compression. Lyytikäinen et al. (2024) have demonstrated that they could produce tablets that are within compendial limits on continuous lines based on formulations developed for batch. However, they did not demonstrate that the same quality could be reproduced nor present data from batch trials. While there have been comparative studies for direct compression, the conclusions are different depending on the experimental conditions. Both Karttunen et al. (2019) and Bekaert et al. (2024) have concluded that CDC resulted in better tablet weight uniformity. Macchietti et al. (2024) presented that no significant differences in API contents were observed between batch and CDC trials despite the running time of the CDC.
However, Karttunen et al. (2019) reported better API content uniformity for CDC, whereas Bekaert et al. (2024) observed that CDC caused a larger variability in API contents due to a lack of feeder optimization for cohesive APIs. These differences are caused due to less consideration of the impacts of formulations and process settings and a lack of statistical analysis, which makes fair comparison difficult. Furthermore, the impact of batch and CDC lines on tablet dissolution has yet to be analyzed.
The current study provides a comprehensive analysis of dissolution behavior of immediate release tablets comparing batch and continuous direct compression methods, using ibuprofen, a BCS Class II API. Design of Experiments (DoE) was performed to assess the impact of blend formulation properties and process settings on tablet dissolution, in addition to the difference in the operation mode. The investigation encompassed both micro- and macro-level perspectives. Initially, dissolution profiles were compared within identical formulation and processing conditions.
Subsequently, a Partial Least Squares (PLS) regression model was constructed using the complete dataset to evaluate the impact of operational modes in relation to formulation attributes and compression variables. Furthermore, a transferability analysis was conducted to validate the predictive capacity of batch trial data on the dissolution behavior of tablets manufactured via a continuous direct compression line.
Read more here
Materials
Formulations contained 25 % of Ibuprofen (Ibuprofen 50, BASF SE, Germany) combined with a selected filler combination, croscarmellose sodium (Ac-Di-Sol®, FMC, Philadelphia, USA) as a disintegrant, silica (Aerosil 200 Pharma, Evonik, Hanau-Wolfgang, Germany) as a glidant, and sodium stearyl fumarate (PRUV®, Moehs Cantabra, S.L., Polanco, Spain) as a lubricant. The filler combinations consisted of microcrystalline cellulose (MCC, Avicel® PH102, DuPont, Cork, Ireland) with dibasic calcium phosphate.
Kensaku Matsunami, Alexander Ryckaert, Hanne Verrecas, Ana Filipa Tavares da Silva, Andrew Anderson, Håkan Wikström, Melanie Dumarey, James Mann, Thomas De Beer, Valérie Vanhoorne, Ashish Kumar, Comparative analysis of tablet dissolution behavior: Batch vs. Continuous direct compression, International Journal of Pharmaceutics, 2025, 125498, ISSN 0378-5173, https://doi.org/10.1016/j.ijpharm.2025.125498.
Read also our introduction article on Sodium Stearyl Fumarate here:
