Complexities related to the amorphous content of lactose carriers
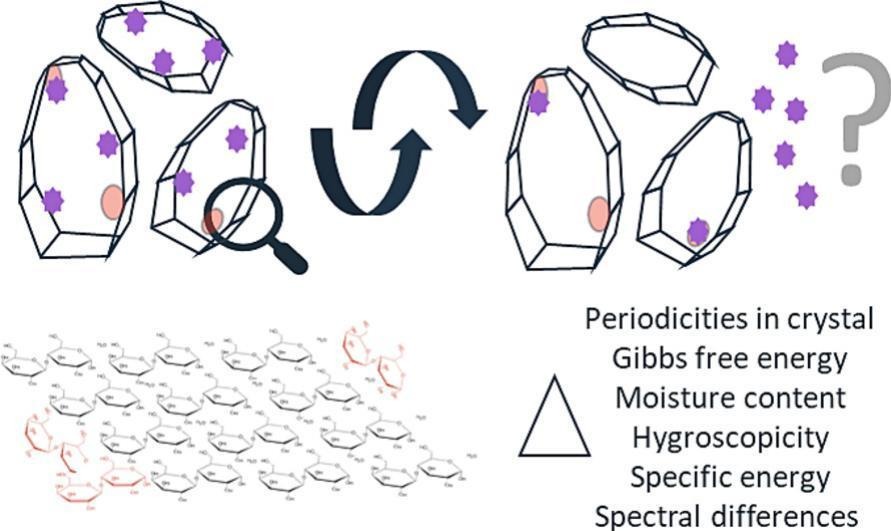
Abstract
Although the amount of amorphous content in lactose is low, its impact on the performance of a dry powder inhalation formulation might be high. Many formulators and regulatory agencies believe that the levels of amorphous content should be controlled once there is a relationship with the final product performance. This is however not an easy task. The current paper elaborates on multiple challenges and complexities that are related to the control of the amorphous content in lactose. The definition and quantification methods of amorphous lactose are reviewed, as well as challenges related to thermodynamic instability. Additionally, current monographs and recent position papers considering this parameter are discussed to provide an overview of the regulatory landscape. Development of a control strategy is recommended, provided that the amorphous content at a specific moment in the process has shown to have an impact on the performance of the dry powder inhaler.
Highlights
- The attention of regulatory agencies and formulators to amorphous content has increased.
- The apparent amorphous content in lactose is difficult to define.
- Different quantification methods can result in different values.
- The apparent amorphous content can be unstable over time.
- Control strategies should only be developed when the amorphous content has an effect on the final product performance.
1. Introduction
Apparent amorphous lactose is a collective term used for all phases of lactose that do not have a defined crystalline structure. Over the last years, the apparent amorphous content of inhalation grade lactose gained interest from the pharmaceutical industry (FDA, 2018; Moreton et al., 2020). Many formulators and regulatory agencies believe that the levels of amorphous content should be controlled, once there is a proven relationship with the final product performance. This is however not an easy task, due to the unstable nature of amorphous and the complexity of quantification techniques.
Many different methods exist to evaluate the apparent amorphous content in a lactose sample. All these methods however, face the challenge that amorphous refers to a broad set of types of disorder in crystal structures with variable material properties (Lehto et al., 2006).
It can be difficult for formulators to determine the apparent amorphous content range that leads to acceptable product quality. This is, amongst others, related to the unstable character of amorphous lactose. The amorphous content in lactose is thermodynamically unstable due to the high energy associated (Huppertz and Gazi, 2016; Wijayasinghe et al., 2020). Dependent on the temperature and humidity, amorphous lactose is susceptible to crystallization. Besides the unstable character of amorphous lactose, performing structured analyses is challenging as samples that only vary in amorphous content are hard to get. Apparent amorphous content is not an independent parameter, and thus steering on this parameter in isolation is hardly possible.
The current monographs for lactose described in the European Pharmacopeia (EP), United States Pharmacopeia- National Formulary (USP-NF), Japanese Pharmacopeia (JP), Indian Pharmacopeia (IP) and the Chinese Pharmacopeia (CP) do not describe any mandatory testing for the apparent amorphous content (Lactose Hydrate Monograph, 2022; Lactose Monohydrate Monograph, 2021; Lactose Monograph, 2022; Lactose Monohydrate Monograph, 2020; Lactose Monohydrate Monograph, 2023). The parameter is however in scope of many discussion on future regulatory frameworks, including position papers and the US FDA draft guidance for industry (Moreton et al., 2020; Lactose for inhalation for CP2025, 2022).
All in all, there are many complexities associated with the apparent amorphous content in lactose. It is a challenging parameter that has the attention of the industry, and an in-depth understanding and clear agreements between drug authorization holders and suppliers is critical.
This article elaborates on multiple complexities associated with the apparent amorphous content in inhalation grade lactose. Section 2 introduces carrier-based dry powder inhalation (DPI) and the importance of interaction forces between an active pharmaceutical ingredient (API) and a carrier. Different polymorphic forms of lactose, including the amorphous form and its thermodynamically unstable character, are introduced. Section 3 evaluates the influence of amorphous content on the performance of lactose carriers. In section 4 the complexities and differences between different methods used to quantify the apparent amorphous content are reviewed. Section 5 describes Quality by Design and control strategies to mitigate the risk of variation in performance of dry powder inhalation as a result of variation in the apparent amorphous content. Chapter 6 reviews the regulatory landscape related to the amorphous content in inhalation grade lactose. The section highlights the current pharmacopeial standards and proposed changes on amorphous content in lactose for inhalation. Section 7 concludes the previous sections.
2. Carrier-based dry powder inhalation
Dry powder inhalers (DPIs) are compact and portable devices that are designed to deliver medication in the form of a dry powder to the lungs. Pulmonary delivery is non-invasive and patient-friendly. It provides a rapid and predictable onset of action, due to the large surface area of the lungs that is available for absorption (Anderson et al., 2022). Additionally, lower doses can be required to reach the local target effective dose, due to the avoidance of the first-pass effect of the gastrointestinal tract and the liver (Patton et al., 2004; Chen et al., 2020).
2.1. Formulation
The efficacy of DPIs relies on the deposition of the API into the lungs, which is predicted in-vitro by the aerodynamic particle size distribution (APSD). The APSD defines how powders behave in an airstream during inhalation. It depends amongst others on the size, shape and density of particles. The optimal aerodynamic particle size to reach the bronchial region is below 5 μm, with the majority of the particles being 2–3 μm (Peng et al., 2016). Crystalline API particles are therefore generally too large and require size reduction, like can be achieved with micronization. Micronized particles however, have a high surface area and high surface energy. Hence high cohesive and adhesive forces are present, resulting in flowability and dispersibility challenges. The most common approach to address these challenges is to formulate the micronized API with a carrier excipient. The carrier has coarser particles than the API, typically between 50 and 100 μm in diameter, and forms a loose bond with the drug (Peng et al., 2016).
Drug delivery is initiated by a quick single inhalation event by a patient through the device, which actuates the dispersion of the formulation into the inhaled air. The energy of the inspired air flow drives the detachment of the drug particles from the surface of the carrier, so drug particles can be deposited into the lungs. Successful drug delivery by carrier-based DPIs depends on many factors. These factors include amongst others the device, the filling platform, the drug or active pharmaceutical ingredient (API), the carrier and the blending process (De Boer et al., 2012). The balance between adhesive and cohesive forces should be adjusted to a level that enough adhesion is present to manufacture a stable formulation, yet easy separation is allowed during inhalation (Pilcer and Amighi, 2010). The interaction forces between drug and carrier are typically dominated by physical forces. These forces include van der Waals forces, electrostatics, capillary forces, and interlocking (Telko and Hickey, 2005). The magnitude of these forces varies with many factors like particle size, shape, surface properties, and contamination of the carrier particle and relative humidity (Hickey et al., 2007).
2.2. Lactose as a carrier
Lactose is the most widely accepted carrier for DPI formulations in the market (Hebbink et al., 2022; Rahimpour and Hamishehkar, 2012). The advantages of using lactose as a carrier are numerous. Lactose is a natural, soluble excipient with good physical and chemical stability (Villalobos-Hernández and Villafuerte-Robles, 2001). It has a long historic track-record resulting in a large set of (favorable) toxicology and safety data (Baldrick and Bamford, 1997). Additionally, it is well accepted by authorities and it is available with well controlled particle size and flow properties. This versatility of lactose can be exploited to optimize each formulation towards the desired performance (Hebbink et al., 2022; Pilcer et al., 2012). Extrinsic addition of lactose fines to a coarse lactose carrier for example, significantly enhances the aerosolization performance and delivery of the API (Kinnunen et al., 2014).
Marketed inhalation lactose grades typically consist mostly of α-lactose monohydrate, which is the most stable polymorph of lactose. Other crystal structures, like β-anhydrous lactose, stable anhydrous α-lactose and hygroscopic anhydrous α-lactose however also exist (Kirk et al., 2007). The different polymorphs differ in properties like stability, hygroscopicity, crystal structure, shape, surface energetics, and chemical reactivity (Kirk et al., 2007; Della Bella et al., 2016). Amorphous lactose is a collective term that is used for all phases of lactose that do not have a defined crystalline structure. It is therefore a thermodynamic unstable phase that encompasses many different types of structural disorder, each with its own properties (Price and Young, 2004). Structural disorder could for example vary in the frequency and scale of crystal disruptions (Peltonen and Strachan, 2020). Additionally, local disorder could be present as a result of anisotropic lattice distortions, amorphous surface layers, mutarotation, particle size reduction disruptions and crystal defects (e.g. vacancy, vacancy dislocation, substitutional impurity, interstitial dislocation loop, dislocation, interstitial self-atom, interstitial impurity) (Garnier et al., 2008; Föll, 2019).
Even though marketed inhalation grades are classified as α-lactose monohydrate, small fractions of amorphous lactose in these materials cannot be excluded. The crystalline state of lactose monohydrate can be inadvertently rendered amorphous by the introduction of varying degrees of stress (Newman and Zografi, 2014). Stresses that can generate layers of amorphous lactose include stresses from drying and comminution operations (Bronlund and Paterson, 2004; Savolainen et al., 2010). Amorphous surface layers that originate from drying processes are categorized as type I apparent amorphous lactose. Type I amorphous lactose layers typically form during the crystallization process of lactose. Adhering solvents after filtration may cause dissolved lactose to remain at the surface of the crystals. During drying, these lactose molecules do not get the time to crystallize and therefore will appear in a completely random structure, that is referred to as an apparent amorphous domain (Dominici et al., 2022). Down-stream sieving, milling or micronization processes of lactose monohydrate could also result in apparent amorphous domains (typically denoted as type II apparent amorphous). In this case, the energy that is applied to reduce the size of lactose particles disrupts the crystal phases of the molecules (Pazesh et al., 2017; Badal Tejedor et al., 2018). Fig. 1 shows a graphical representation of these two types of amorphous lactose in a lactose monohydrate crystal. It is worth highlighting that the different types of amorphous lactose are not always very distinct – and also intermediates or different types of disorder can exist.
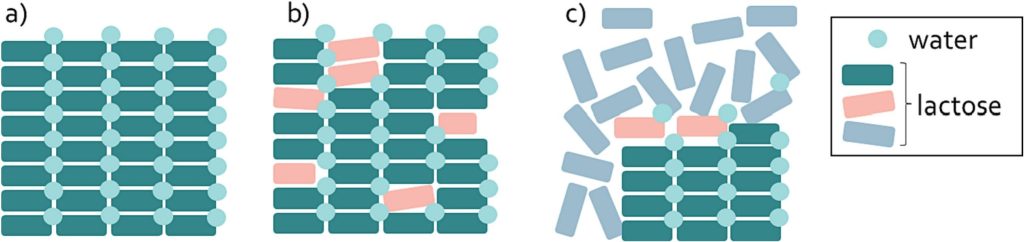
2.3. The unstable character of amorphous lactose
Amorphous lactose is thermodynamically unstable in nature due to the high energy associated with this form. There is a thermodynamic drive to reduce the energy of the system typically leading to recrystallization (Huppertz and Gazi, 2016). This recrystallization is highly impacted by internal and external conditions. These factors include the type of amorphous structure (Haque and Roos, 2005), the presence of impurities (Wijayasinghe et al., 2020), the temperature and the relative humidity (Clark et al., 2016). Packaging, transport and intermediate opening of packaging could play a crucial role in the amount of crystallization. The predictability of the speed and amount of crystallization is therefore typically very low. Fig. 2 shows an example of recrystallization of two lactose grades with different initial amounts and types of apparent amorphous lactose.
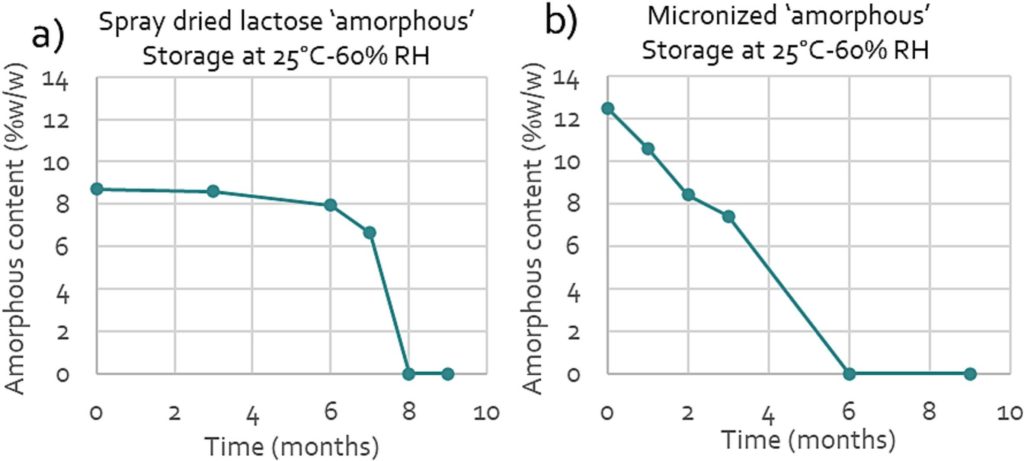
Both graphs show a decrease in the apparent amorphous content over time, due to recrystallization. Based on this observation, it becomes clear that the amorphous content is a material property that is variable over time. Therefore, drawing relationships between the amorphous content and the performance of lactose can only be done based on actual data on the apparent amorphous content available. This can only be achieved when the determination of amorphous content is performed at the time that the material is used in the formulation. Data on the apparent amorphous content directly after production of a raw material is not representative as it does not reflect the amorphous content upon use.
Download the full article as PDF here Complexities related to the amorphous content of lactose carriers
or read it here
Pauline H.M. Janssen, Lorina M.N. Bisharat, Marly Bastiaansen, Complexities related to the amorphous content of lactose carriers, International Journal of Pharmaceutics: X, Volume 6, 2023, 100216, ISSN 2590-1567, https://doi.org/10.1016/j.ijpx.2023.100216.