Fused Deposition Modelling 3D printing and solubility improvement of BCS II and IV active ingredients – A narrative review

Abstract
In the field of pharmaceutical research and development, Fused Deposition Modelling (FDM) 3D printing (3DP) has aroused growing interest within the last ten years. The use of thermoplastic polymers, combined with the melting process of the raw materials, offers the possibility of manufacturing amorphous solid dispersions (ASDs). In the pharmaceutical industry, the formulation of an ASD is a widely used strategy to improve the solubility of poorly soluble drugs (classified by the Biopharmaceutical Classification System (BCS) as class II and IV). In this review, an analysis of studies that have developed a FDM printed form containing a BCS class II or IV active substance was performed. The focus has been placed on the evaluation of the solid state of the active molecules (crystalline or amorphous) and on the study of their dissolution profile. Thus, the aim of this work is to highlight the interest of FDM 3DP to induce the amorphisation phenomenon of Class II and IV active substances by forming an ASD, and as result improving their solubility.
Introduction
The oral route is the preferred manner for drug administration due to its simplicity and acceptability. Nevertheless, the formulation of oral pharmaceuticals is more complicated for poorly soluble active substances. Indeed, after oral administration, an active pharmaceutical ingredient (API) will have to be solubilised in the gastrointestinal tract in order to be absorbed and reach the systemic circulation, therefore the less soluble the API is, the less bioavailable it is. Yet, it appears that 40% of marketed drugs and up to 90% of molecules under industrial development are poorly soluble [1,2]. Under the supervision of the Food and Drug Administration (FDA), the Biopharmaceutical Classification System (BCS) divides molecules according to their solubility and permeability; thus while Class I and III include highly soluble molecules, poorly soluble molecules are classified as Class II (poorly soluble, highly permeable) or IV (poorly soluble, poorly permeable) [3,4]. Given the clinical interest of several molecules belonging to the Class II and IV, it is essential to find new strategies to improve their solubility [5]. Several methods of formulating Drug Delivery Systems (DDS) have been developed to overcome this problem, such as the addition of solubilisers (complexing agents, surfactants), the use of the salt-form of molecule drug, lipid-based formulation, nanotechnologies, micellar systems, micronisation, solid dispersion, etc. [6,7]. Among these techniques, solid dispersion and in particular amorphous solid dispersion (ASD) have been widely used to improve solubility and thus the bioavailability of drugs [8].
The amorphous state of an API is characterised by a conformational disorganisation of the molecules, in contrast to the crystalline form, where the molecules are arranged in symmetrical crystal arrays [9]. Amorphisation is produced when a phase transition defined by high molecular mobility is reached. Amorphous materials are characterised by a glass transition temperature (Tg), below which they are in a rigid/solid state and above which they are in a rubbery state with increased molecular mobility. Molecular mobility must be carefully assessed because if it is too high, at temperature above Tg the molecules could bind and form nucleation points, initiating recrystallisation. As the amorphous form tends to return to a more stable crystalline state, the dispersion of the API amorphous form in a polymeric matrix, will contribute to maintain it by preventing the molecules to bind together, and thus recrystallizing. Several manufacturing processes have been reported to produce ASD, these methods are mainly based on solvent evaporation, precipitation and mechanical breaking or cooling of the melt [10,11]. In recent years, new methods of production have been developed, such as 3D printing (3DP). 3DP is an additive manufacturing technique of three-dimensional objects. The principle lays in adding successive layers of material to reproduce a 3D digital model created with a computer-aided design (CAD) software [12]. Recently, significant effort is being made to implement 3DP techniques in the pharmaceutical field due to its interest for personalized treatments [13]. Research and development in the field of 3DP of medicaments have highlighted the possibility of obtaining different DDSs with the ability to modulate API release, drug loading, size, shape, taste, and even texture [14,15]. Together, these elements contribute to improved acceptability and finely tailor patient adapted forms. Other perspectives might include producing printed forms of new drug entities that are not yet available, or designing polypills containing several active substances in order to reduce the frequency of administration [16,17]. Moreover, the implementation of 3D printers within a drug preparation unit in hospitals and/or pharmacies would enable the production of small, customised batches of the drugs responding to a clinical interest [18,19].
There are different 3DP techniques available on the market, and among them, Fused Deposition Modelling (FDM) 3DP, is the most widely used in pharmaceutical applications due to its accessibility and precision [20]. FDM 3DP is based on the melt extrusion of a polymer-based intermediate product (IP). The feeding of the IP into the 3D printer can be done in two different ways. The most common method is to load the raw material in the form of a filament obtained by a previous Hot Melt Extrusion (HME) process [21,22]. The HME process is already used in the pharmaceutical industry for the development of DDS-type solid dispersions [23]. This process involves the compression and passage of material through a die, the diameter of which determines the dimensions of the resulting product. The raw materials are introduced through the main or secondary feed hoppers and are conveyed into the screw assembly. The barrel is the outer cylindrical part containing the screw system. The temperature inside the system is regulated to ensure the melting of the raw materials. The rotating screw system helps to provide the energy needed to soften the materials and ensure a homogenous mixture. The IP exits at the downstream end of the die, in the form of a filament [21,23]. Once produced, the filament passes through a heated nozzle of the printer which melts the filament before deposition. This process is referred as “Fused Filament Fabrication” (FFF) 3DP.
Alternatively, in the case of the IP being in powder form, the technique is called 3DP Direct Powder Extrusion (DPE). This approach consists in loading a powder directly into the printer and melting it before depositing it on the printing surface [24]. The deposition of the molten material, layer by layer, reproduces the transmitted digital 3D model.
Other 3DP techniques, based on the same fusion principle, can be included in the FDM process such as Hot-Melt Pneumatic Extrusion (HPME) or even Arburg Plastic Free-forming (APF). The HPME is similar to the DPE technique in that the raw material in powder or granulated form is introduced directly into the system. However, in HPME, the melt is necessarily extruded using a pneumatic system [25]. In the case of APF, the raw material in pellets form is fed from the hopper and passes through a single screw extruder (similar to HME process) where the heating takes place. Then, the molten material is pushed into the heated print-head to be deposited on the printing surface [26].
In all cases, the primary mixture in powder form is composed of a blend of the API and polymers. The polymers have thermoplastic properties and are involved in the formation of a matrix dispersing the API during the melting processes. There is then, one or two heating steps depending on the raw material used in FDM 3DP (respectively DPE/HPME or FFF/APF), during which the temperatures applied and polymer addition are conducive to the creation of an ASD.
Thus, in this review the great prospects of using FDM 3DP for improving the solubility of Class II and IV APIs by ASD formation are presented. Furthermore, other relevant considerations related to the storage and stability of the FDM printed forms are also addressed.
Read more here
Agnès Roche, Noelia M. Sanchez-Ballester, Bernard Bataille, Violaine Delannoy, Ian Soulairol, Fused Deposition Modelling 3D printing and solubility improvement of BCS II and IV active ingredients – A narrative review, Journal of Controlled Release, Volume 365, 2024, Pages 507-520, ISSN 0168-3659, https://doi.org/10.1016/j.jconrel.2023.11.041.
Read more on “Pharmaceutical 3D printing” here:
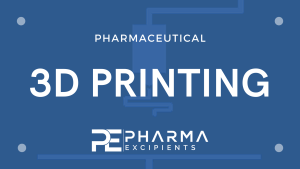