3D Printing Direct Powder Extrusion in the Production of Drug Delivery Systems: State of the Art and Future Perspectives
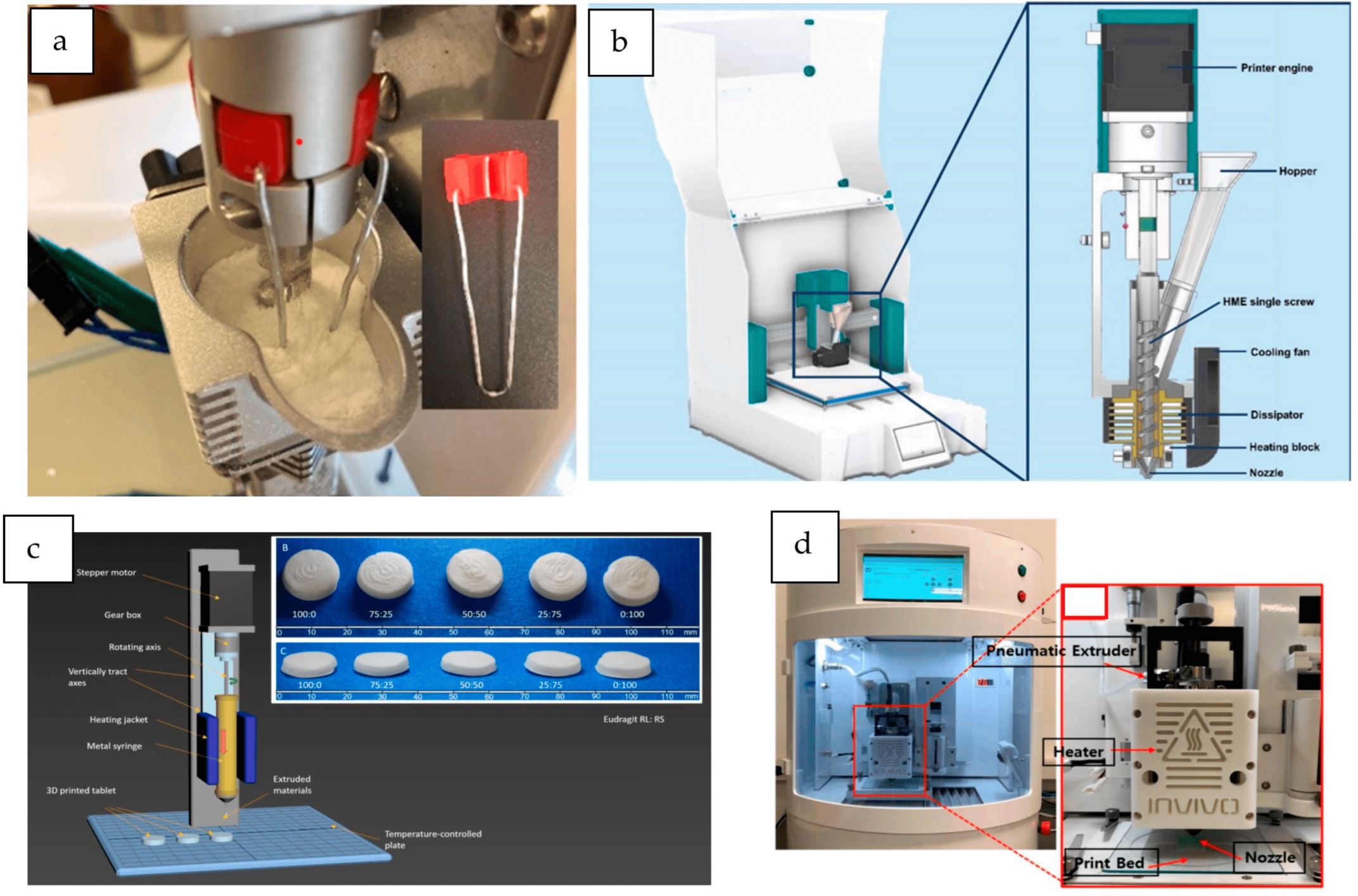
Abstract
Introduction
Three-dimensional printing (3DP) has been introduced as an innovative and versatile technology to manufacture drug delivery systems due to its precision and accuracy, efficiency, personalized prescription, and ability to create complex and customized dosage forms. Extrusion-, powder-, and laser-based techniques are the main categories of 3DP techniques, with fused deposition modeling (FDM) and semisolid extrusion (SSE) being the most commonly used extrusion-based techniques in pharmaceutical development. FDM involves the extrusion of a filament obtained from a mixture of thermoplastic polymers, drugs, and/or auxiliary excipients. It allows the production of solid dosage forms with customized doses [1], release profiles [2], or geometries [3,4], and a combination of different drugs [5,6], which demonstrates its efficacy in the context of personalized medicine [7]. It can also be highlighted by the cost-efficiency and wide range of printers and materials used. However, FDM presents several limitations, such as the need for filaments (usually obtained by hot melt extrusion (HME)) with appropriate mechanical and physical properties (homogeneous diameter and adequate stiffness and brittleness), the double heating and consequent thermal stress of the pharmaceutical ingredients, and the tendency of amorphous drug molecules to recrystallize during storage [8]. For all the above reasons, the process of filament optimization is time-consuming, and there is a limitation in the choice of drugs and excipients.
SSE is an alternative technique based on the extrusion of highly viscous liquids or semisolid materials (gels, pastes, or solids with a relatively low melting point) from pre-filled syringes at room or moderate temperatures, making it more suitable for thermolabile drugs. However, a post-printing solidification process is often required. The complete solvent removal in some cases and the risk of material collapse and loss of shape are other drawbacks of this technique [9,10].
A new material extrusion 3DP technique called direct pellet extrusion (DPE) was introduced in 2017 as a promising alternative to FDM printing in the plastics industry [11]. This technique involved the extrusion of pellets or powders previously melted in a “melt generation unit” through the die of a single-screw extruder to the nozzle of the printer to be directly printed without the previous preparation of filaments using HME.
This way, the equipment necessary to process the formulations is reduced [12]. In addition, mixtures that cannot be printed by FDM due to the inappropriate mechanical properties of the filaments obtained could be potentially extruded using this technology. In DPE, the material flow towards the printer nozzle is mainly driven by the screw rotation, which is slightly influenced by the material’s mechanical properties [13,14,15,16]. Moreover, the thermal stress experienced by the APIs processed by DPE is significantly reduced in comparison with FDM [17,18,19].
Additionally, unlike HME, which usually requires high amounts of excipients to avoid producing too brittle or too flexible filaments to be loaded into an FDM printer, this technique overcomes such a limitation [18], showing its ability to print systems with high drug loadings even without additional excipients [16].
Another important advantage of this technique is the small amount of powder mixture required, which is useful when a small quantity of a drug is available, as in the case of the preclinical studies [20].
The objective of this systematic review is to analyze the application of DPE techniques to the manufacture of drug delivery systems. An overview of the 3D DPE printers used, the critical parameters of the formulation and printing process, and the drug delivery systems produced with this technique is carried out. Finally, future perspectives for the implementation of this technology in healthcare centers are discussed.
3.3.1. Oral Administration
The most commonly used geometrical shape for the manufacturing of oral dosage forms by DPE is a simple cylinder. Nevertheless, this 3D printing technology can lead to complex internal designs, resulting in structures such as core–shell and multi-compartment [8,14,15,16,20,22,23,24,26,28,29,30,34,35]. These modifications allow the combination of different drugs in the same dosage form and/or modulation of drug release profiles, as described in the following section.
- 3D-printed tablets
Immediate drug release tablets
Immediate drug release dosage forms can be successfully prepared by the DPE technique. The heating of components and their rapid solidification often entail a change from crystalline to amorphous forms of API, as confirmed by X-ray diffractograms and DSC results. This change leads to a higher solubility and dissolution rate of the drug.
One of the most commonly used polymers for this technique is Kollidon® VA64, a water-soluble copolymer of N-vinylpyrrolidone and vinyl acetate (monomer ratio 6:4), also known as copovidone. Its low Tg (100 °C) makes it suitable for DPE, although it often requires the addition of a plasticizer. Recently, this polymer has been used to prepare immediate-release cylindrical tablets containing efavirenz (5–35% w/w) for the treatment of HIV [29]. The efavirenz granulates were formulated with Kollidon® VA64 (40% w/w) and mannitol (20–55% w/w) and magnesium stearate (5% w/w) as plasticizers and were used as the feeding material for DPE. The printed systems released more than 80% of drug loads within the first 60 min.
Copovidone has also been used in combination with the copolymer poly vinylalcohol-polyethylene glycol (Kollicoat® IR) to prepare immediate-release 3D-printed tablets containing levodopa (28% w/w) and carbidopa (7.56% w/w) in two doses (60/15 mg and 170/42.5 mg). This combination is of clinical interest in the treatment of Parkinson’s disease [23]. Kollicoat® IR is less brittle than copovidone, and its higher melt viscosity promotes adhesion on the built plate. It also improves the mechanical properties of the printed tablets. The mixture also requires the addition of mannitol (10% w/w) to provide the most reliable printing process and fast tablet disintegration. In this work, the influence of the tablet shape (cylinder, torus, and oblong) (Figure 4a) on the disintegration and dissolution behavior was investigated. The results showed faster dissolution for the oblong tablets due to their reduced infill.
Kollidon® VA64 has also been used in the manufacture by DPE of pediatric cylindrical tablets containing 35 and 50% w/w of praziquantel (100 and 150 mg, respectively) [28]. Surfactants (Span™ 20 or Kolliphor® SLS Fine) were added to the formulations to increase the dissolution of the solid dispersions. Praziquantel is a Class II BCS drug characterized by a low drug solubility (0.02 mg/mL), an unpleasant taste, and variable dosing, great challenges that need to be overcome in the development of a pediatric oral formulation. Tablets were successfully produced from both pellets and powders obtained from HME extrudates. The results indicate that Praziquantel remained amorphous due to the HME treatment before printing, even after 3 months of storage at 25 °C/60% RH. Due to the formation of amorphous solid dispersions, in vitro praziquantel release was clearly improved. Moreover, the formulation of 3D-printed tablets was helpful for taste masking when compared to powder dosage forms.
On the other hand, Kollidon® VA64 has been used in combination with a hydrophilic matrix, such as HPC SSL, allowing milder processing conditions, such as lower temperatures and pressures, compared to the use of HPC as a single excipient. Fanous et al. [39] prepared oval tablets with a honeycomb infill pattern containing 10% w/w caffeine as a model drug. Different proportions of HPC (45–90% w/w), with or without Kollidon® VA64 (31.5% w/w), and PEG 4000 (13.5% w/w) were used. Kollidon® VA64 acts as a rapidly dissolving polymer, facilitating the dissolution process. The research demonstrates the effect of infill density and thus geometry on the release rate, as an increase in surface area results in greater water access and faster dissolution.
Formulations with 65% w/w of Kollidon® VA64 and 5% w/w of PEG 1500 were used to obtain cylindrical tablets containing 25% w/w of metoprolol succinate. Complete drug release was achieved after 60 min [30]. In this work, the acrylic polymer Eudragit® EPO was also tested, confirming its ability to produce immediate-release dosage forms by DPE.
Among the acrylate derivatives, Eudragit® E100 has also been evaluated by DPE to obtain 3D-printed cylindrical tablets. Jennotte et al. [35] used Eudragit E100 and Soluplus® (SOL) (polyvinyl caprolactam–polyvinyl acetate–polyethylene glycol graft copolymer) in an 18–40% w/w range, due to their ability to increase the solubility of poorly soluble drugs, for the preparation of ASDs containing 10% w/w of cannabidiol (65 mg). These two polymers are not printable on their own because of their brittleness, so Polyox® N10 (PEO) was added as a plasticizer (50–72% w/w, depending on the formulation). Cannabidiol was chosen as a BCS II model drug, and dissolution results showed an immediate drug release (80% of the drug released in 45 min) from the printed systems studied.
Some studies compare the behavior of different polymers processed by DPE in order to increase the dissolution rate of a poorly soluble drug in comparison with tablets obtained by direct compression. For instance, Kim et al. [40] prepared four formulations with dutasteride (1% w/w), Lutrol® F 68 (10% w/w), and one of the following polymers (89% w/w): Soluplus®, Kollidon® VA64, Eudragit® EPO, and HPC. The authors managed to enhance the dissolution of the drug, which was in an amorphous state with Kollidon® VA64, reaching above 80% of drugs released within 15 min. HPC also increased the dissolution rate when compared with directly compressed tablets. No significant differences were found for the other polymers. The authors also investigated four different 3D-geometries (cube, pyramid, hemisphere, and tube) to evaluate the effect of the surface area/volume (SA/V) ratio on the drug release profiles. It was demonstrated that the larger the SA/V ratio, the faster the drug release.
Sustained drug release tablets
Prolonged drug release from 3D-printed tablets can be achieved through different strategies. One of the most used is matrix formation using hydrophilic or hydrophobic polymers such as HPC, hydroxypropymethyl cellulose (HPMC), copovidone, EVA, methacrylate derivatives, etc.
Among the hydrophilic polymers, HPC has been widely used in FDM 3D printing due to its suitable mechanical properties and ease of extrusion. Four different low-viscosity grades of HPC have been evaluated as matrix-forming excipients for DPE: HPC-UL (MW 20,000), HPC SSL (MW 40,000), HPC-SL (MW 100,000), and HPC-L (MW 140,000) [20,26]. These soluble polymers were used at a concentration of 65% w/w without any other adjuvant to prepare cylindrical tablets of itraconazole [20]. All the polymer grades were successfully printed, showing good adhesion between the printed layers. In addition, powder X-ray diffraction (PXRD) and DSC results suggested that the drug was amorphous in the formulations. In fact, the dissolution studies revealed a drug concentration 20 times higher than the itraconazole solubility. All printed formulations showed sustained release over more than 24 h, with a zero-order release profile during the first 8 h. It was also observed that the lower the molecular weight of the HPC, the faster the drug release.
HPC-SL and HPC-L in 50–60% w/w were also used to prepare cylindrical tablets containing 5% w/w of tramadol hydrochloride with alcohol-resistant and abuse-deterrent properties. Formulations with 50% w/w of HPC also contained 40% w/w D-mannitol and 5% w/w magnesium stearate, while formulations with 60% w/w of HPC contained 20% w/w PEO, 10% w/w D-mannitol, and 5% w/w magnesium stearate [26]. A prolonged-release profile over 12 h was observed due to the formation of a thick viscous gel layer. The X-ray diffractograms of the tablets revealed no crystalline peaks corresponding to tramadol, suggesting that the drug was in an amorphous state. All the formulations exhibited alcohol-resistant and moderate abuse-deterrent properties, meeting the objectives of the study. This technique was advantageous when compared to other methods of production of this type of formulation, such as injection molding, which implies high costs in the production of reduced batches.
Another hydrophilic polymer employed in DPE is polyvinylpyrrolidone (PVPK12). Lee et al. [41] used this polymer (75% w/w) with PEG 1500 (5% w/w) to prepare customizable modified-release solid dosage forms of ibuprofen (20% w/w) as a model API. Different shapes, such as cylinders, rings, and a floating dosage form with a cavity, and the fusion of two different geometries in the same dosage form were evaluated (Figure 4b). Diffractogram results showed that ibuprofen was molecularly dispersed in the extrudates in an amorphous form.
HPMC HME 15 LV is used as a water-soluble cellulosic polymer to enhance the solubility of poorly soluble drugs by maintaining stable solid dispersions and inhibiting API crystallization. Moreover, it has a polymer substitution structural design that facilitates thermal processability by HME, making this excipient an excellent candidate to produce stable ASDs with poorly soluble APIs. Thus, formulations containing 10% w/w of niclosamide (50 mg) and HPMC HME 15 LV (40–90% w/w) were processed by DPE to obtain cylindrical tablets (see Figure 4c) [14]. The effect of the addition of 4.5% w/w of PEG 6000 to the previous formulations was also studied. The resulting systems showed a prolonged and complete drug release over 48 h, following zero-order release kinetics during the first 8 h. The presence of hydroxypropyl-β-cyclodextrin (HP-β-CD) (47% w/w) in the mixture was also investigated to improve the poor water solubility of niclosamide.
The results show that the drug release from the dosage forms occurred through the matrix swelling and solubilization, since the combination of HPMC and HP-β-CD showed a synergistic effect improving the aqueous solubility of the drug. Furthermore, DSC, Fourier transform infrared spectroscopy (FT-IR) and PXRD results confirmed the amorphization of the drug during the printing process, which also contributed to the increase in drug solubility. The amorphous state of the drug is maintained after 3 months of storage at 25 °C and 60% RH [14].
Copovidone has also shown the ability to produce sustained-release tablets by DPE. Liu et al. [38] used a mixture of copovidone (56% w/w), acetaminophen (35% w/w), and the plasticizer Poloxamer® 407 (9% w/w) to prepare sustained-release cylindrical tablets of three different commercial strengths (650 mg, 500 mg, and 350 mg).
On the other hand, hydrophobic polymers such as EVA are also used as matrix polymers. Almeida et al. [46] used EVA without any other adjuvant excipient to produce sustained-release dosage forms via HME. This fact, along with the flexible physicochemical properties of this polymer, makes it a potential candidate to be employed with hot-processing techniques such as DPE. The physical and mechanical properties of the copolymer differ with the VA content, being rigid at low VA contents and rubbery at high VA contents. The 3D-printed cylindrical tablets containing metoprolol tartrate (50% w/w) and EVA with different VA content (50% w/w) were manufactured by DPE [34]. All pellet formulations were successfully printed, unlike filaments, which could only be printed by FDM in the case of EVA with 18% VA content due to mechanical and rheological problems. Feuerbach et al. [12] were also able to process formulations containing 100% of EVA (28% VA content) in powder form with a homemade hot-end adapted to a conventional FDM printer, despite its unsuitability in filament form [36]. Therefore, it seems that the mechanical and rheological properties of the raw materials are less limiting factors for DPE. All tablets showed high content uniformity and a homogeneous distribution of metoprolol, which remained crystalline in the EVA matrix. A prolonged drug release profile over 12–24 h was observed for all preparations.
Poly(meth)acrylates, such as Eudragit® RL and Eudragit® RS, are other hydrophobic polymers that have also been used to produce sustained-release cylindrical tablets by DPE. Kuźmińska et al. [24] prepared different formulations containing theophylline (40% w/w) and varying the proportion of Eudragit® RL and Eudragit® RS (0–40% w/w). It was possible to modulate the theophylline release profile by changing the methacrylate polymer ratios. The release rate of theophylline decreased as the Eudragit® RS content increased due to the lower hydrophilicity of this polymer. The formulations required an excess of the plasticizer triethyl citrate (TEC) (12%), chosen by its miscibility with the acrylate polymers, in combination with glycerol monostearate (8%), to be extruded. The use of these plasticizers and lubricants also allowed the blends to be printed at lower temperatures (up to 110 °C maximum).
Finally, poly (3-hydroxybutyrate) (PHB) has also been investigated as a promising alternative biopolymer for DPE [33]. This thermoplastic aliphatic polyester is biodegradable, biocompatible, and environmentally friendly, with a high crystallinity (60–70% w/w) and a molecular weight > 400 kDa. The authors prepared three different model square-shaped systems with acetaminophen as the model drug (10, 20, and 30% w/w). Dissolution results showed a prolonged release for these systems, following a diffusion process.
- Core–shell 3D-printed tablets
Core–shell tablets are a common design in 3D printing technology, particularly in FDM [47,48,49]. This structure offers the possibility to modulate the drug release profile by only changing the characteristics of the drug-free shell, such as the thickness or composition, without modifying the size of the core containing the drug [50]. Recently, DPE has been used to fabricate core–shell geometry controlled-release tablets containing acetaminophen (40% w/w) in the core [8]. Acetaminophen was chosen as a model drug due to its compatibility and its ability to form hydrogen bonds with polymers, making it widely used in the production of solid dispersions. Moreover, it is thermally stable at temperatures below 260 °C. During printing at 140 °C, acetaminophen (Tm 172 °C) was converted from its crystalline to the amorphous state, being dissolved in molten Kollidon® VA64. This polymer was used as the core filler (60% w/w) due to its water solubility and its application in the manufacture of ASDs. The drug dissolution profiles showed a complete drug release from the core tablet in 5 h.
The immediate-, sustained-, and delayed-release polymers Eudragit® E PO, Eudragit® RS PO, and HPMCAS LG were used as shell polymers. Eudragit® E PO is a copolymer of dimethylaminoethyl methacrylate and other neutral methacrylic acid esters that dissolves at pH < 5 and has great printability. Eudragit® RS PO is a copolymer synthesized from acrylic acid and methacrylic acid esters, widely used for extended release due to its low permeability and water-insoluble character. HPMCAS LG is a mixture of acetic acid and monosuccinic acid esters of HPMC in granular form, often used as an enteric coating due to its pH-sensitive character (soluble at pH > 5.5) and rapid dissolution in the upper intestine. It is also useful in the formulation of ASDs. HPMCAS LG exhibits a high melt viscosity, being necessary a plasticizer for processing. Hence, PEG 4500 was added as a plasticizer agent (20% w/w) in formulations containing HPMCAS LG and as a pore-forming agent (10–20% w/w) in those based on Eudragit® RS PO.
As expected, core–shell tablets with pure Eudragit® E PO showed a lower drug release rate than the core tablet, but the dissolution of the shell and the core was not sequential, and water uptake into the inner core was facilitated by the interlayer spaces of the shell. The presence of PEG 4500 in the HPMCAS LG shell formulation increased its porosity, and these tablets exhibited an extended rather than delayed release. Coating with Eudragit® RS PO and PEG 4500 was more effective to protect the core, showing these systems zero-order drug release kinetics over 20 h. The authors demonstrated that the dissolution rate could be modulated by varying the shell composition.
- 3D-printed minitablets
Minitablets are small tablets, typically with a diameter lower than 4 mm. The small size of the tablets offers several advantages, as they are easy to swallow and commonly accepted by children [51]. They also offer the possibility of being incorporated into a hard capsule, where different formulations can be combined.
DPE 3D printing has been used to manufacture 3D-printed spherical minitablets containing 25% w/w of either ritonavir or lopinavir (40 mg) to develop a personalized solid dosage pediatric formulation for HIV treatment [18]. HPMCAS LG grade (51.75% w/w) was used as the matrix forming polymer. Other components of the formulation were the plasticizer PEG 4000 (22.5% w/w) and the lubricant magnesium stearate (0.75% w/w). Hydrogen bonding interactions between drugs, HPMCAS and PEG 4000, resulted in the production of ASDs with a sustained-release zero-order kinetics profile at pH 6.8. The fraction of the drugs dissolved remained in solution despite their low solubility in the intestinal pH. The minitablets showed high uniformity in drug content, weight, diameter, and density.
The fabrication of these dosage forms was rapid, and the minitablets produced could be fitted in a size 0 hard capsule. They can be easily swallowed by pediatric patients, allowing a versatile administration of the drug adapted to their weight. An accurate dose can be achieved by changing the diameter of the minitablet or the number of minitablets administered.
In another study, cylindrical minitablets loaded with budesonide and intended for the pediatric treatment of eosinophilic colitis were manufactured by DPE [15]. Three different formulations containing 0.59% w/w of budesonide (1 mg) were prepared. The results obtained demonstrated the amorphization of the drug during the printing process. HPMC HME 15 LV (Affinisol®), a cellulose derivative with thermal properties suitable for extrusion-based 3D printing processes, was used as the matrix polymer in a 41–75% w/w, depending on the formulation. All the mixtures included an adjuvant blend (8–20% w/w) consisting of sodium bicarbonate, citric acid, and tartaric acid to accelerate the HPMC dissolution and prevent the drug recrystallization in the gel layer. HP-β-CD (47% w/w) was added to improve the solubility of the active ingredient, and PEG 6000 (2.99–3.97% w/w) was used as a plasticizer. The time required to complete the printing process of each tablet was approximately 3 min, and the minitablets obtained showed good mechanical and physical properties, as well as a sustained-release profile. To achieve a colon-specific drug release, the minitablets were coated in a fluidized bed with Eudragit® FS30D.
Cylindrical minitablets containing nifedipine for the treatment of hypertension were also prepared by DPE [16]. The drug loading of each printed system was 25% w/w (20 mg). Other components of the formulation were HPC LF (40% w/w) and HPMCAS LG (19% w/w) as rate-controlling polymers, PEG 4000 (15% w/w) as plasticizer, and magnesium stearate (1% w/w) as lubricant. The amorphization of the drug was observed after the printing process, and a prolonged drug release profile based on erosion was obtained over 24 h. This release profile was preferable to that exhibited by commercially available formulations that disintegrate quickly, showing a pronounced burst effect. The printing time of each tablet was 2 min, and the dose could be easily adjusted to the patient’s needs by simply modifying the tablet height, facilitating the production of personalized medicines. The minitablets obtained can be fitted inside a 0-size capsule with other minitablets containing other active ingredients, which would allow the preparation of a multidrug solid dosage form. A minimal risk of physicochemical interactions between drugs is expected, as each drug is contained in an independent tablet.
- Multicomponent tablets
Multicomponent tablets offer the possibility of incorporating different drugs with different release kinetics, even if they show incompatibility issues, independently in the same dosage form. Multimaterial printing also allows the design of different structures within the dosage form. In this sense, compartmental-model tablets with six different designs were prepared by melt extrusion deposition (MEDTM) employing a GMP-compliant (MEDTM) 3D printer with multiple printing stations [22]: multilayered drug compartment in core–shell structure tablets, modified release tablets in core–shell structure with a delay layer, modified release tablets in core–shell structure with a pH-responsive layer, multi-compartment tablet for independent release of drug combinations, multi-component tablet for concurrent release of immediate-release formulation and extended release formulation and single-compartment tablet for sequential release of immediate-release formulation and extended release formulation. Different model drugs (metoprolol succinate, tofacitinib citrate, levodopa and carbidopa, topiramate and clonidine hydrochloride) and a wide range of polymers (Eudragit® RS PO, HPC EF and JF, HPMCAS HG and LG, ethyl cellulose (EC), and Kollidon® VA64) and auxiliary excipients (PEG 400, PEG 1500, PEG 8000, stearic acid, TEC, and croscarmellose sodium) were employed. It was demonstrated that the direct 3D printing of compartmental designs allowed the modulation of the release onset time, release kinetics, and release duration with high precision and reproducibility. Furthermore, the authors of this study proposed a product development approach called 3D printing formulation by design (3DPFbD®) to offer a simple and effective tool for the rapid and efficient development of pharmaceutical dosage forms.
Download the full article as PDF here
or read it here
Aguilar-de-Leyva, Á.; Casas, M.; Ferrero, C.; Linares, V.; Caraballo, I. 3D Printing Direct Powder Extrusion in the Production of Drug Delivery Systems: State of the Art and Future Perspectives. Pharmaceutics 2024, 16, 437. https://doi.org/10.3390/pharmaceutics16040437
Are you interested in our webinar:
“Rethinking the development of controlled release formulations and manufacturing processes”
Date: 30th of April, Time: 3:00 pm (Amsterdam, Berlin)