3D printed capsule shells for personalized dosing of cyclosporine-loaded SNEDDS
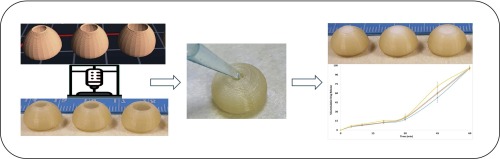
Abstract
Cyclosporine (CsA) is a potent immunosuppressant agent that has been used since 1980 for the treatment of various autoimmune diseases and is extensively used to enhance the survival rate of patients and grafts following organ transplant surgeries. CsA is a poorly soluble drug with a narrow therapeutic window and inter-subject variability, which can lead to graft rejection, nephrotoxicity and other severe adverse effects. This study explores a novel method that combines solubility enhancement of CsA using SNEDDS formulation and personalized dosage delivery using 3D printing technology. The oil phase was chosen as a combination of caproyl 90 and octanoic acid while the Smix phase was chosen as a combination of cremophore El and PEG 400. The optimized liquid SNEDDS was solidified using PEG 6000. An FDM printer was used to print a capsular shell with an oval base that ascends to form a dome with an opening at the top. This opening is used to fill the molten CsA-loaded SNEDDS formulation using a pipette or syringe. The CsA-loaded SNEDDS formulation was characterized by FTIR, DSC and SEM/EDX. The in-vitro release of CsA showed complete release within sixty minutes and followed Korsmeyer-Peppas release kinetics. The drug release was not affected by either the shell opening size or the amount of the loaded formulation. This novel method is simple and straightforward for personalized dosage delivery of drug-loaded SNEDDS formulations.
Introduction
Cyclosporine (CsA) is a cyclic polypeptide, lipophilic in nature, and a US-FDA-approved primary immunosuppressive agent used to reduce the rejection rate in organ transplants and for the treatment of several autoimmune diseases (Tedesco and Haragsim, 2012). Despite its therapeutic efficacy, CsA is associated with limitations such as poor biopharmaceutical characteristics (high molecular weight, poor aqueous solubility, high lipophilicity, low intestinal permeability, and susceptibility to CYP3A-mediated metabolism) that lead to incomplete oral absorption and high inter-subject variability contributing to drug-associated nephrotoxicity (Tedesco and Haragsim, 2012, Patel and Wairkar, 2019).
Various approaches have been explored to address CsA’s poor properties, leading to improvement in therapeutic efficacy and reduced toxicity (Al-Lawati et al., 2018). Sandimmune® and neoral® from Novartis exemplify to enhance CsA’s solubility and absorption, ultimately increasing its bioavailability and therapeutic efficacy. Both products utilize the self-emulsifying drug delivery system (SEDDS), but they differ in the droplet size produced upon self-emulsification: Sandimmune® generates microemulsion (SMEDDS), while Neoral® generates nanoemulsion (SNEDDS) with droplets of size between 100–250 nm. This reduction in droplet size significantly improves CsA’s bioavailability (Gursoy and Benita, 2004).
SNEDDS involve the solubilization of poorly aqueous soluble drugs in an isotropic formulation mixture composed of a lipid phase (such as oils) and a Smix phase (a combination of surfactants and co-surfactants). This mixture, when administered orally and mixed with GI fluids forms nanoemulsions (Jain et al., 2015, Bang et al., 2019). Encapsulating the active therapeutic in nano-size oil droplets enhances overall oral absorption. However, liquid SNEDDS as exemplified by Neoral®, face stability and compatibility issues, and the formulation may leak from capsules (Kohli et al., 2010, Thomas and Rades, 2013). Consequently, researchers are actively exploring methods to transform liquid SNEDDS, preserving the advantages of improved drug solubility and bioavailability while eliminating the stability and compatibility drawbacks of liquid SNEDDS (Tang et al., 2008).
Common techniques for transforming liquid SNEDDS into solid formulations include adsorption to solid carriers, wet granulation, spray drying and extrusion/spheronization (Mandić et al., 2017). However, optimizing suitable additives, solid carriers and process parameters is crucial for each solidification technique to maintain the self-nano emulsification characteristics (Mandić et al., 2017, Kang et al., 2012).
A growing interest has emerged in 3D printing technology as a novel platform to address complex pharmaceutical challenges that cannot be resolved using conventional methods (Mohammed et al., 2021, Mazarura et al., 2022). This interest was particularly piqued following the FDA’s approval of the first 3D-printed tablet of levetiracetam (SPRITAM®) in 2015 (FIRST FDA-APPROVED MEDICINE MANUFACTURED USING 3D PRINTING TECHNOLOGY, 2023). Recently, our research group developed a tablet dosage form of Dapagliflozin-containing SNEDDS using PAM-based 3D printing technology for oral administration. This approach enabled us to enhance drug solubility and produce tablets in various sizes by altering printing dimensions facilitating drug dispensing tailored to individual dose requirements (Algahtani et al., 2021). Another research group, led by Aikaterini-Theodora Chatzitakiet employed PAM-based 3D printing technology to design and develop lidocaine containing SNEDDS as a suppository for patient-specific lidocaine therapy for local anaesthesia. The mechanical properties and release profile of the printed suppositories adhere to European pharmacopoeia standards (Chatzitaki et al., 2021).
Here we present a more clinically applicable method for dispensing SNEDDS formulations in individualized doses that healthcare practitioners can prepare without extensive 3D printing expertise. A novel FDM-printed shell design allows the shell to be filled with the molten formulation and subsequently surrounds the solidified content for enhanced formulation protection. This approach enables the filling of the required dose or a series of doses in a single step using a simple pipette. CsA is utilized as a model drug in this study due to its aforementioned poor solubility and narrow therapeutic window. Moreover, there is a frequent need for dose adjustment, particularly after organ transplantation surgeries, to achieve therapeutic efficacy and prevent adverse reactions or body rejection of the allografts (Lindholm and Kahan, 1993, Hu et al., 2006).
Read more here on 3D printed capsule shells for personalized dosing
Materials
Cyclosporine A (CsA, purity 99 %) was purchased from TCI Chemicals, UK. Capryol 90 was obtained from Gattefosse, France. PEG 400 was purchased from UFC Biotechnology NY, USA. Octanoic acid, Cremophor EL, PEG 6000, Acetonitrile (HPLC grade), and Methanol (HPLC grade) were purchased from Sigma Aldrich, UK. Millipore deionized water was used for all experimental work.
Mohammed S. Algahtani, Abdul Aleem Mohammed, Javed Ahmad, Raisuddin Ali, Ehab Saleh, 3D printed capsule shells for personalized dosing of cyclosporine-loaded SNEDDS, International Journal of Pharmaceutics, Volume 650, 2024, 123707, ISSN 0378-5173, https://doi.org/10.1016/j.ijpharm.2023.123707.
Read more on “3D Printing” here:
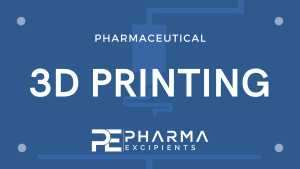