Sugars and Polyols of Natural Origin as Carriers for Solubility and Dissolution Enhancement
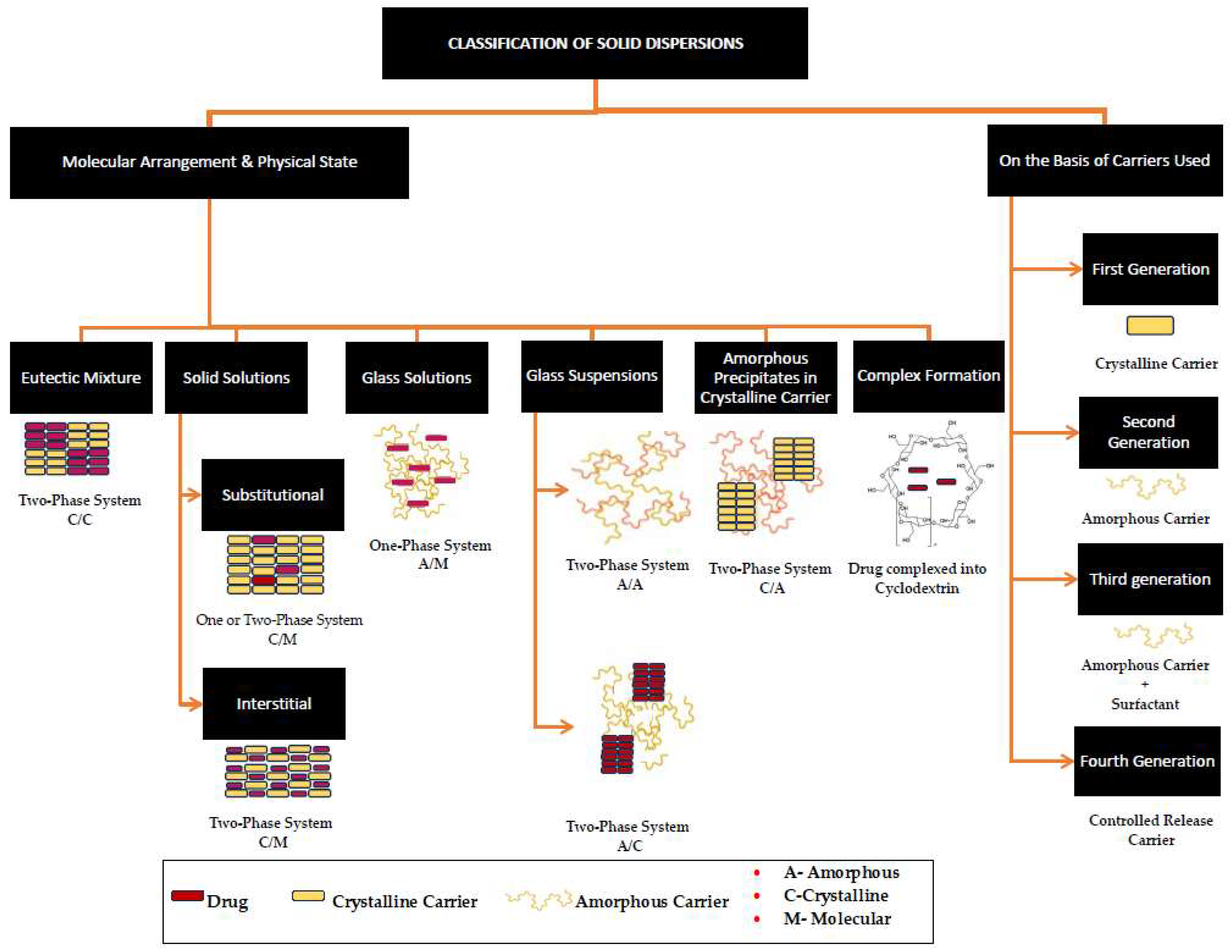
Abstract
Crystalline carriers such as dextrose, sucrose, galactose, mannitol, sorbitol, and isomalt have been reported to increase the solubility, and dissolution rates of poorly soluble drugs when employed as carriers in solid dispersions (SDs). However, synthetic polymers dominate the preparation of drugs: excipient SDs have been created in recent years, but these polymer-based SDs exhibit the major drawback of recrystallisation upon storage. Also, the use of high-molecular-weight polymers with increased chain lengths brings forth problems such as increased viscosity and unnecessary bulkiness in the resulting dosage form. An ideal SD carrier should be hydrophilic, non-hygroscopic, have high hydrogen-bonding propensity, have a high glass transition temperature (Tg), and be safe to use. This review discusses sugars and polyols as suitable carriers for SDs, as they possess several ideal characteristics. Recently, the use of low-molecular-weight excipients has gained much interest in developing SDs. However, there are limited options available for safe, low molecular excipients, which opens the door again for sugars and polyols. The major points of this review focus on the successes and failures of employing sugars and polyols in the preparation of SDs in the past, recent advances, and potential future applications for the solubility enhancement of poorly water-soluble drugs
Introduction
Poor aqueous solubility is a major concern for new chemical entities (NCEs) and many of the existing active pharmaceutical ingredients (APIs) developed for oral drug delivery, where these substances have great pharmacological and permeability potential. An increasing proportion of potential new drug candidates (40–70%) are classified as Biopharmaceutical Classification System (BCS) Class II or IV drugs, and the formulation and commercialisation of these as oral dosage forms presents great challenges as they show sub-optimal dissolution; absorption; and, hence, bioavailability [1]. The poor aqueous solubility has a negative effect on dissolution, resulting in the incomplete absorption of these APIs in the gastrointestinal tract; hence, the bioavailability is affected detrimentally [2,3]. Several techniques are used to overcome this problem, which include physical and chemical modifications of the drug through micronization, crystal engineering, salt formation, the addition of surfactants, solid dispersions with hydrophilic carriers, complexation, hydrotropy, eutectic mixtures, and amorphous systems, to mention but just a few [2,4]. Among these solid-state alterations, solid dispersions and co-amorphous and co-crystal systems have taken the lead over the past two decades. One of the common factors among these techniques that plays a significant role in solubility and bioavailability enhancement is the type of carrier, co-former, or excipient used [5,6]. In the selection of a carrier, preference is given to carriers that are hydrophilic in nature [5], have high hydrogen bonding propensity, have higher glass transition temperatures (Tg), are non-hygroscopic [7], and form part of the United States Food and Drug Administration (FDA)-approved Generally Recognized as Safe (GRAS) list [8].
Naturally occurring sugars of several types, such as monosaccharides (glucose, fructose, galactose); disaccharides (sucrose, maltose, lactose); and sugar alcohols (polyols) such as sorbitol, mannitol, xylitol, erythritol, maltitol, lactitol, and isomalt, have been reported to enhance the solubility of drugs. The FDA designates sugars and polyols as GRAS excipients [9,10], and in addition to their proven safety, these compounds exhibit good water solubility [11] and possess hydroxyl groups (-OH) attached to each carbon atom in the molecule, which, in theory, provides hydrogen-bonding sites for drug molecules, thereby already meeting three of the abovementioned criteria in terms of suitable solid-state co-formers.
There are several ways in which sugars and polyols have been used to enhance the solubility of drugs. One predominant method used is to form solid dispersions of the drug [12]; another approach is the formulation of eutectic mixtures [13,14]. Polyols such as xylitol [15,16], mannitol [15], and sorbitol [17] have been reported as potential co-formers for developing co-crystalline systems. However, efforts made in exploring sugars and polyols in the making of co-crystals and co-amorphous systems are few to none. The low molecular weight, safety, good glass-forming ability, and (in some instances) high Tg of sugars [18] make them an interesting option to explore as excipients for drug–excipient co-amorphous and co-crystalline systems. The current review focuses on summarising the use of naturally occurring sugars and polyols for the enhancement of solubility and the dissolution rate of poorly soluble drugs. Furthermore, it seeks to understand the pros and cons in relation to the various techniques used; to provide a theoretical basis for their application in the formulation of co-amorphous and co-crystalline systems; and finally, to identify potential opportunities for future research.
Table 2. Summary of SDs reported in the literature obtained by using various sugars as carriers.
Method | Sugar | Drug: Sugar (w/w) | Drug | Solubility | Dissolution | Remarks | Ref |
---|---|---|---|---|---|---|---|
Fusion | Dextrose Fructose Maltose | 1:3, 1:1, and 3:1 | Clotrimazole | Fructose showed a slight increase at a 1:1 ratio | Increased dissolution rate for fructose SDs. Increased with an increase in sugar concentration. | Partial dispersion of drug at molecular level. | [7] |
Dextrose Galactose Sucrose | 1:33 and 1:40 | Corticosteroids | N/A | Increased dissolution rate with bi-phasic drug release. | Partial dispersion of drug at molecular level. Hygroscopic and heating resulted in discolouration. | [35] | |
Sucrose–mannitol (1:1) Sorbitol–mannitol (1:1) | 1:19 | Corticosteroids | N/A | Increased dissolution rate with bi-phasic drug release. Galactose showed smaller dissolution rate. | Sucrosemannitol eutectic showed less hygroscopicity and no discolouration. | [36] | |
Lactose Galactose | 1:3 | Carbamazepine Nitrazepam | N/A | Increased dissolution rate with bi-phasic drug release. Galactose showed slower dissolution rate. | Partial dispersion of drug at molecular level. | [63] |
|
Dextrose Icing Sugar Lactose | 4:1, 2:1, 1:1, and 1:4 | Ibuprofen | N/A | Only 80% of the drug was released within 60 min. | An increase in the sugar concentration had an insignificant effect on drug release. | [40] | |
Quench Cooling | Glucose Galactose Maltose Sucrose | 1:1 | Sulfamethoxazole | Sugars with free carbonyl group showed slight increase in solubility. | A 100% drug release in 5 min (glucose and maltose). Galactose showed slower dissolution rate. | Partial dispersion of drug at molecular level. Hygroscopic and heating resulted in discolouration. | [37] |
Lactose | 1:1, 1:3, 1:5, and 1:10 | Carbamazepine Ethenzamide | N/A | Increase in dissolution rate with increase in carrier concentration. Five- to eight-fold increase in dissolution rate. | Hydrogen bonding with amide and carboxyl groups of the CBZ. | [39] |
|
Glucose | 1:0.03 | Indomethacin | N/A | Eight-fold increase indissolution rate. | Ultrasonication of the melt increased the miscibility between the drug and carrier. | [42] | |
Solvent evaporation | Lactose Sucrose | 1:1 and 1:5 | Etoricoxib | 1.5 to 1.8-fold at 1:5 ratio | N/A | Intermolecular hydrogen bonding between S=O group of etoricoxib and O–H group of sugar carriers. | [44] |
G-HCL | 4:1, 2:1,1:1, 1:2, and 1:4 | Carbamazepine | Solubility of solid dispersions is lower than pure drug and physical mixtures | Concentration of carrier and solvent system used affected the dissolution rate | Presence of water in the binary solvent system reduced the dissolution rate because of the formation of the dihydrate form of the CBZ. | [45] |
|
G-HCL | 1:1, 1:2, 1:3, 1:4, and 1:5 | Acyclovir | 12-fold increase | Higher concentrations of carriers (1:4 and 1:5) showed reduced dissolution due to reduced access of the ACV to dissolution medium. | Decreased dissolution rate during storage. Hydrogen bond between amine group of ACV and O-H of G-HCL. | [46] |
|
Freeze drying | Trehalose, Sucrose, InulinDP11 InulinDP23 | Diazepam Nifedipine THC Cyclosporine A | N/A | Solutionmediated phase transition in the case of trehalose, sucrose, and inulinDP11 SDs. | The chain length of inulin affected the Tg of SDs, dissolution behaviour, and stability. | [50,51] | |
Freeze drying followed by vacuum drying | Sucrose α-maltose Trehalose α-lactose | 1:10 | Fat-soluble flavours | N/A | A 100-times increase for α-maltose, trehalose and maltitol in methanol. | ASDs exhibited solutionmediated phase transition after 200 s | [54] |
Trehalose α-maltose Palatinose | 1 to 10% w/w | Indomethacin, Ibuprofen, Gliclazide, Nifedipine | 20–1000% increase | Palatinose and/or α-maltose showed superior dissolution. | ASDs exhibited solutionmediated phase transition because of low Tg. | [55] | |
Trehalose α-maltose Palatinose | 0.1 to 10% w/w | Curcumin | N/A | α-maltose and trehalose showed superior dissolution | ASDs exhibited solutionmediated phase transition. | [56] | |
Kneading | Lactose Maltose Sucrose | 1:1, 1:3, and 1:5 | Allopurinol | N/A | No significant increase in dissolution rate. | No intermolecular interactions found. Slight increase in dissolution rate due to partial amorphization. | [58,59] |
Roller compaction | Lactose Maltose | 1:4 | Griseofulvin | N/A | A 35- to 40-fold increase. | Processing problems due to sticking and physical instability | [61] |
Centrifugal spinning | Sucrose | 1:9 | Olanzapine Piroxicam | Increase in solubility is proportional to sucrose concentration. | A 3- and 1.7-fold increase in dissolution rate of olanzapine and piroxicam, respectively. | Intermolecular interactions observed with olanzapine SDs. No solutionmediated phase transition was observed after 4 h of dissolution. | [62] |
Download the full article as PDF here: Sugars and Polyols of Natural Origin as Carriers for Solubility and Dissolution Enhancement
or continue reading here
Poka, M.S.; Milne, M.; Wessels, A.; Aucamp, M. Sugars and Polyols of Natural Origin as Carriers for Solubility and Dissolution Enhancement. Pharmaceutics 2023, 15, 2557. https://doi.org/10.3390/pharmaceutics15112557