The era of digital pharmacy. 3D printing – realities and perspectives
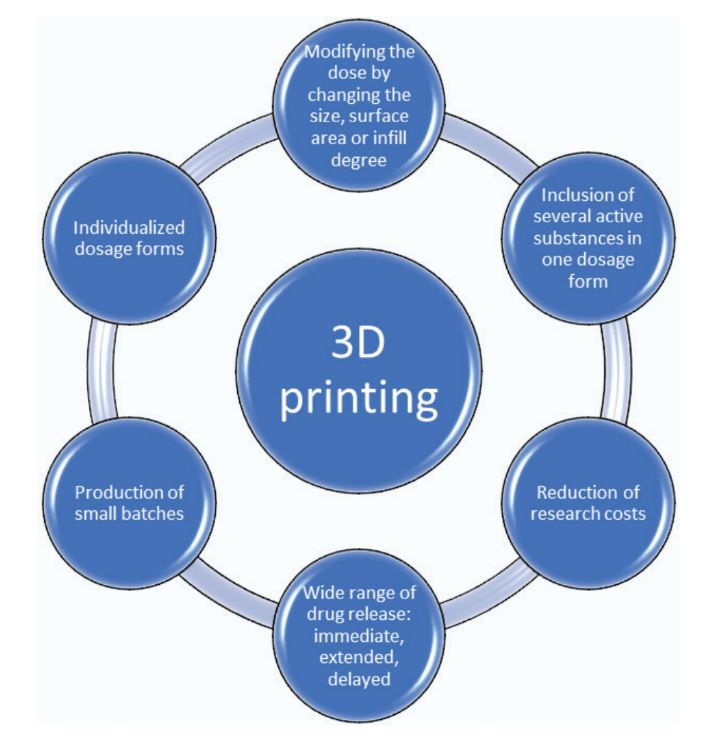
3D printing is an innovative technology for building three-dimensional objects by laying down successive layers of material under the control of a computer software. It is entering pharmacy mainly because of its revolutionary potential to provide individualized dosage forms that meet the needs of each patient, due to the possibility to produce objects of many different sizes and shapes. An important aspect of personalized 3D tablets is the possibility to include several active substances in one dosage form, which would reduce the daily number of medications and the frequency of their administration and improve patient compliance. Another advantage of 3D printing is the possibility of producing small batches or even individual drugs for each patient. Despite the many advantages, 3D printing has several technological challenges to overcome before it becomes widely applicable in pharmacy. Five basic technologies are currently applied in pharmaceutical practice: powder-based printing, selective laser sintering, stereolithography, extrusion moulding printing, and electrohydrodynamic 3D printing. This article reviewed development, research focus, and prospects of each technology respectively.
Introduction
The three-dimensional (3D) printing is an innovative technology for building three-dimensional objects by laying down successive layers of material under the control of a computer software. A main feature of this method is the possibility for production of complex geometric shapes. For this reason, it has already steadily entered the engineering sciences and is about to revolutionize medicine and pharmacy (Alhnan et al. 2016).
The terms “three-dimensional printing”, “additive printing”, “additive manufacturing” and “rapid prototyping” are used as synonyms for 3D printing. In conventional manufacturing methods, the desired shape of the object is achieved by removing excess material from a monolithic block, hence the retronyms “subtractive manufacturing” and “subtraction technology”. “3D printing” is used to describe various methods in which objects are built layer by layer, hence the term “additive manufacturing” (Norman et al. 2017; Roopavath et al. 2017).
In summary, the 3D printing process proceeds as follows: the object to be printed is drawn three-dimensionally using Computer Aided Design (CAD) software and exported as a stereolithographic image (stl. file). This file “cuts” the 3D object into a series of 2D images that the printer prints on top of each other to create the specified complex 3D object. Therefore, the main components of 3D printing technology can be divided into three groups: 1) hardware (the 3D printer itself); 2) software (to communicate with the hardware and to convert the images drawn by CAD into stl. files that are recognized by the printer, and 3) materials used for printing (Roopavath et al. 2017). 3D printing is entering pharmacy mainly because of its revolutionary potential to provide individualized dosage forms that meet the needs of each patient (Gaisford 2017). The need for personalized medicine emerges from the extremely complex structure of the human organism. Various factors such as age, sex, health status and expression of certain genes influence the pharmacokinetics and pharmacodynamics of drug molecules. For this reason, different individuals require different dosage regimens or dosage forms, which are not always available (Awad et al. 2018). This is notably valid for paediatric and geriatric patients. Dosage requirements in these groups change dynamically, in the former due to rapid changes in the physiological and metabolic functions of the body, and in the latter due to pathologies in the gastrointestinal tract and renal clearance. In elderly patients, polypharmacy and comorbidities should also be considered (Alomari et al. 2015). Until now, various methods of personalized dosing have been used in pharmaceutical practice, but the paradigm of individual drugs, in which the dose, the dose combination or the active substance itself are tailored to the patient’s genetic profile, is not yet fully understood (Gaisford 2017).
Of course, pharmaceutical technology has so far offered a variety of mechanisms for individualizing the dose. One of the classic approaches is the use of liquid dosage forms – individual dosing can be easily accomplished by taking different volumes, usually with the help of a dosing device included in the product packaging. However, the risk of dosing inaccuracy should not be overlooked. This also applies to the most common approach for solid dosage forms – splitting “scored” tablets in half. There is also data about modern approaches such as dosing devices for multiparticulate pellet systems, Solid Dosage Pen, and oral films that can be cut into individual segments allowing for individual dosing (Wening and Breitkreutz 2011; Alomari et al. 2015).
An approach for individualizing the dose is also inkjet printing that can be considered a precursor to 3D printing. The idea generated from printers that recreate digital images on paper by dripping tiny droplets of ink. The technology has been adapted for pharmaceutical purposes as the ink is replaced by a solution of an active substance and the paper – by edible sheets called substrates (Alomari et al. 2015). The dose variation can be achieved by changing the number of printed layers for a given area or by increasing the print area itself. One of the advantages of this technology is the good control over the precision of the dose combinations and the release of the active substance. It is very suitable for the production of low-dose drugs, especially those with doses in the range of micrograms (Alomari et al. 2015; Alhnan et al. 2016).
3D printing is a very promising platform for individual dosing because, compared to the approaches described so far, it has the flexibility to produce objects of many different sizes and shapes. This advantage is the subject of scientific research aimed at modifying the dose by changing the size, surface area or the infill degree of the printed tablet. This is especially necessary for paediatric patients, which are characterized by both large variations in prescribed doses and swallowing difficulty (Alhnan et al. 2016). If 3D printing is combined with 3D scanning technology that captures the anatomical features of each patient, it can be used for individualized medicinal products (Muwaffak et al. 2017; Liang et al. 2018). Studies are published describing pre-scanned products printed according to the shape of the patient’s nose (Goyanes et al. 2016; Muwaffak et al. 2017), as well as dental protectors made from a combination of polylactic acid and polyvinyl alcohol (Liang et al. 2018), and intrauterine implants (Genina et al. 2016; Holländer et al. 2016).
An important aspect of personalized 3D tablets is the possibility to include several active substances in one dosage form, which would reduce the daily number of medications and the frequency of their administration and improve patient compliance (Khaled et al. 2015a). A number of studies have been published related to this topic, for example tablets, containing combinations of chlorpheniramine maleate and diclofenac (Katstra et al. 2000), rifampicin and isoniazid (Genina et al. 2017), or paracetamol and caffeine (Goyanes et al. 2015b). The therapeutic efficacy of fixed-dose combinations in cardiovascular disease is well established. However, applying these combinations, individual patient characteristics and needs may be overlooked. In this regard, 3D dosage forms containing three (captopril, nifedipine and glipizide) (Khaled et al. 2015a), five (hydrochlorothiazide, ramipril, acetylsalicylic acid, pravastatin, and atenolol) (Khaled et al. 2015b) active ingredients would be appropriate for application.
In addition to dose variation, different types of additive printing also demonstrate a wide range in drug release. On the one hand, powder-based technology achieves revolutionary rapid disintegration. An example of this is the first FDA-approved 3D printed drug product, Spritam, produced using the company’s patented Aprecia ZipDose technology. On the other hand, by Fused deposition modelling (FDM) technology could be achieved immediate (Arafat et al. 2018; Ehtezazi et al. 2018) or extended (Goyanes et al. 2015c; Pietrzak et al. 2015) and delayed (Goyanes et al. 2015d; Genina et al. 2017) drug release. Another advantage of 3D printing is the possibility of producing small batches or even individual drugs for each patient. The compact design and simplified software of some printer models make them suitable for positioning close to where pharmaceutical care takes place (in hospital and open-label pharmacies) (Alhnan et al. 2016). This would allow personalized e-prescriptions to be fulfilled in pharmacies (Awad et al. 2018). On the other hand, the rapid production of small batches of medicinal products and medical devices would significantly reduce research costs (Goyanes et al. 2018) (Fig. 1).
Despite the many advantages, 3D printing has several technological challenges to overcome before it becomes widely applicable in pharmacy. For example, in extrusion methods and powder-based technology, the construction of successive layers of material is carried out by means of nozzles. Their clogging has a negative impact on the reproducibility of the process (Alhnan et al. 2016; Norman et al. 2017). In powder-based technology and selective laser sintering, the removal of the remaining non-bonded material from the powder bed must also be considered, therefore this type of printer can only be located in special premises (laboratories or production facilities) but not in open-type pharmacies (Alhnan et al. 2016). For technologies involving the presence of a solvent (extrusion of semi-solid material and powder-based technology) an additional drying stage is also necessary, which leads to prolongation of the work process. In addition, both methods yield fragile structures that do not meet the pharmacopoeial requirements for tablet friability (Yu et al. 2009). Certain limitations in the choice of excipients must also be considered. For example, only photopolymerizable oligomers (Fuh et al. 1999) can be used for stereolithography, and thermoplastic polymers must be used for Fused deposition modelling (Awad et al. 2018).
Download the full article as PDF here: The era of digital pharmacy. 3D printing – realities and perspectives
Dimitrov M, Georgieva D, Ilieva S, Petkova V (2023) Te era of digital pharmacy. 3D printing – realities and perspectives. Pharmacia 70(4): 1083–1092.
https://doi.org/10.3897/pharmacia.70.e111731
See the webinar:
“Rational Selection of Cyclodextrins for the Solubilization of Poorly Soluble Oral Drugs”, 8. November 2023:
Get more information & register here for free:
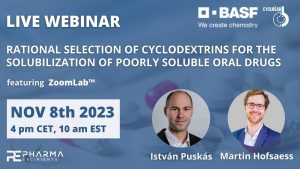