PLGA – A versatile copolymer for design and development of nanoparticles for drug delivery
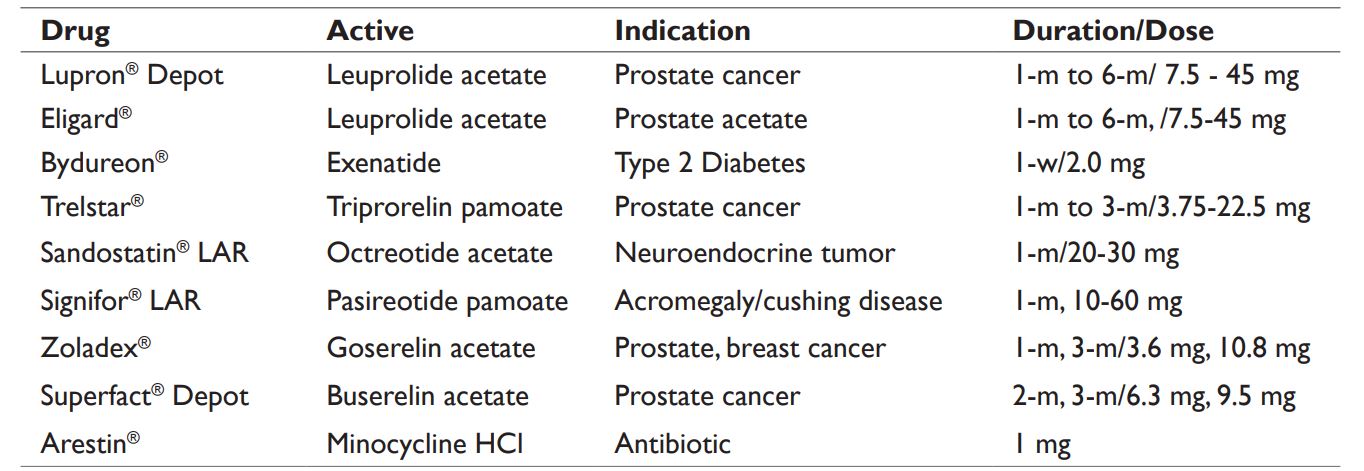
Introduction
Poly (lactic-co-glycolic acid) or PLGA, a highly hydrophobic copolymer comprised of lactic acid and glycolic acid, has been approved in many drug products and medical devices.1 Over the years since the first approved in 1997, PLGA has been widely studied polymer in the industry because of its compatibility and safety, especially in oncology.2 As a homopolymer of two polymerized acidic moieties, one relatively hydrophobic lactic acid and other hydrophilic glycolic acid. PLGA with its unique LA/GA composition ratios and molecular weight provides a good barrier to protect the remature release and degradation of drugs encapsulated in the microspheres or nanoparticles. Because of its biocompatibility and biodegradability in nature, it has also been used in several oncology drugs.3
This article will focus on the chemistry, properties, applications and the regulatory aspects of PLGA and the future trends in the industry especially those requiring the development of long acting injectable of treatment for varieties of rare diseases and for life cycle management.
Structure of PLGA
It is synthesized by ring opening chemistry that follows the copolymerization of monomer of lactic acid and glycolic acid moieties. PLGA is polyester where these moieties are linked together by ester linkages to a linear aliphatic polymer as shown in Figure 1.

and y = glycolic acid units).
Different grades of PLGA are commercially available for pharmaceutical applications as microspheres, nanoparticle and medical devices.
Depending upon the molar ratios of lactic acid and glycolic acid used in the synthesis, the PLGAs used for pharmaceutical applications are comprised of 75% lactic acid and 25% glycolic acid and/or 50% each of lactic acid and glycolic acid. PLGA with its lactic acid and glycolic acids molar ratios of 75:25 or 50:50 or 85:15, exists in an amorphous state.4 PLGA with higher GL accelerates the degradation as compared to lower GA and higher LA moieties. For example, PLGA with 50:50 shows faster degradation than PLGA with 65:35, 75:25 and 85:15 GA/LA due to inherent hydrophilicity of glycolic acid.5
The glass transition temperature (Tg) ranges 40 o
C to 60 o C depending upon the molecular weight (MW), and its solubility in organic solvents depends upon the lactic acid/glycolic acid ratios.
PLGA is typically soluble in a wide range of chlorinated methylene chloride. chloroform, and hexafluoro isopropanol (HFIP) and nonchlorinated solvents such as THF, acetone, DMSO, glycofural, ethyl acetate, ethanol, N-methyl pyrrolidone, methyl ethyl ketone, acetonitrile, isosorbid dimethyl ether, dioxane among others.6 In water, PLGA biodegrades by hydrolysis of its ester linkages. The methyl side group in poly lactic acid moiety makes it relatively more hydrophobic than polyglycolic acid and hence lactide rich PLGA copolymers are
less hydrophilic and susceptible to degradation by hydrolysis.5 Faster degradation depends on the ratios of the 2 acids; higher the lactic acid, slower the degradation and vice versa. In LA/GA 1:1 copolymer, the degradation is fast, leading to formation of lactic acid and glycolic
acid within 3 months. Lactic acid is metabolized in tricarboxylic acid cycle and gets excreted via carbon dioxide and water, whereas, glycolic acid metabolized the same pathway as lactic acid, and it gets excreted through kidney. Glycolic acid is also metabolized into oxalic acid, which may lead to systemic toxicity in body during implant or longer circulation of PLGA microsphere.
Characteristic and drug delivery of PLGA microspheres
Physicochemical properties of PLGA such as molecular weight, LA:GA copolymer ratios, crystallinity, nature of drug and its loading as well as the particle size, morphology, porosity of the nanoparticles may affect the in vitro drug release characteristics.7 The challenges in utilizing PLGA stems from encapsulating hydrophilic drugs into hydrophobic PLGA. The challenges with reducing burst release and increasing encapsulation efficiency of hydrophilic drugs in PLGA require additional functional hydrophilic layers. To overcome this issue, additional functional layers, like hydrophilic gels/hydrogels around the PLGA core–shell microspheres for sustained release. Yu et al. demonstrated losartan loaded PLGA microspheres with gel cores along the exteriors yielded high encapsulation efficiency of watersoluble drugs.8 By selecting 5% gelatin in the inner core, release was over 30 days as compared to 16 days release with water in the inner cores. With 25% Poloxamer 407 as hydrogel, it formed showed initial slower release but as P407 swelled, microcapsules cracked that resulted in faster release of drugs. In other cases where Poloxamer 407 hydrogel can encapsulate the small molecules like goserelin, a hydrophilic peptide, and acts as hydrogel depot at the exterior PLGA depot encapsulated with the same drug prepared by double emulsion solvent evaporation method with combined encapsulation efficiencyof >94%. The resulting di-depot structure with PLGA and Poloxamer 407 showed 2 weeks controlled release, first diffusion of drug through PLGA core followed by diffusion of drug through Poloxamer 407 core.8
Porosity of PLGA microspheres
The single layer core is dense and non-porous for delivery of hydrophilic or hydrophobic drugs, it may lead to slower release due, in part, to slower erosion of polymer via hydrolysis. These challenges can be alleviated by reducing the polymer concentration and varying polymeric chain lengths of LA and GA moieties with different molecular weight and/or by selecting the appropriate preparation methods designed with different outer surfaces. Those modifications
include hydrophilic shell comprised of exterior polyethylene glycol moieties exposed to water, and a lecithin layer is sand-witched between the two, that prevents the degradation of polymer chain and controls the erosion from water, whereas, the exterior PEG layer help stabilize the structures by creating the electrostatic charges with zeta potential of 40 mV to -60 mV around the microspheres ranging between 60 nm and 70 nm in size. An example includes, docetaxel
prepared with PLGA-lecithin-PEG core with 62% encapsulation efficiency, and release kinetics was controlled by diffusion of drug through lipid layer.9
As compared with tradition PLGA single layered core, the porous microspheres have also been used as a reservoir for extended release of drugs. Mahboubian et al. for instance, evaluated an anti-cancer drug triptorelin from porous PLGA microspheres prepared by double emulsion process prepared with 2% Poloxamer 407 in first emulsion solution (w/o) containing drug and PLGA in methylene chloride that was emulsified by homogenization before mixing with 0.5% polyvinyl alcohol to form w/o/w double emulsions.10 The release kinetics from porous PLGA microspheres (130µ in size) were about twice faster as compared to PLGA without Poloxamer 407 (110µ in size). The encapsulation efficiency with Poloxamer 407 decreased by 7% because of its ability to occupy the hydrophilic surface and competing with drug, hence, allowing a lesser degree of encapsulation in PLGA microspheres. 11 Varga et al. also observed the impediment of higher loading of vitamin E due to competing Poloxamer 407 stabilizer at the non-porous, smooth PLGA icrosphere with hydrophilic surface prepared by nano-precipitation in acetone followed by lyophilization. Increasing amounts of Poloxamer 407 resulted in larger particle size (ca. 212 nm) with drug loading (DL) of 9% and encapsulation efficiency (ee) of 41% as compared to ee of 98% ee and DL of 20% DL with smaller particle size (ca. 178 nm) at 0.05% poloxamer 407 as stabilizer.12
PLGA microsphere’s core shells are distinct in nature, and depending upon the usage of these nanoparticles, they may have varying core shapes, internal structures, shell thicknesses and morphologies and porosities.13 The latter is most explored for designing and increasing drug loading in PLGA microspheres for controlling the delivery by changing the porosity of the PLGA cores. Pore size and porosity of PLGA microspheres with broader surface area and lower density play an important role in faster releasing of drugs.14
A number of porogen agents such as sodium chloride, ammonium bicarbonate, a tri-block copolymer Poloxamer 407, sodium oleate, gelatin, bovine serum albumin, cyclodextrins and mineral oils have been investigated for creating porous PLGA microspheres.6 Porosity can be modified by solvents and polymer concentrations. For instance,
solvents with lower boiling points, the pores are larger and vice versa.15 Likewise, increasing the polymer concentrations from 1% to 5%, the porosity decreases about 25%.16 Other porogen agents have also been studied. For instance, Qutachi et al. prepared the porous PLGA microspheres with average size of 84 microns and pore size
of 8µ to 15µ by treatment for 2 min with ethanolic sodium hydroxide (EtOH/NaOH) and used as injectable cell carriers.17 Ni et al., while investigated PVP as porogen agent and stabilizer for higher drug (e.g. cinaciguat) loading to prepare the porous PLGA by o/w emulsion solvent evaporation method for treatment of pulmonary hypertension via inhalation.18 Mylonaki et al reported that the internal structure of atorvastatin (ATV)-loaded PLGA microspheres is dependent upon the MW, glass transition temperature (Tg ) and most importantly on porosity on drug release kinetics, which in turn dependent upon drug characteristics such as melting point, logP and its concentration in the formulation.19 The hydrophobic drugs release through diffusion PLGA matrix whereas, the hydrophilic drugs release through porous route. Shen et al. investigate the drug release from porous risperidone loaded microspheres and compared with regular microspheres.20 For instance, porous microspheres were prepared by dissolving risperidone
in benzyl alcohol at 24% and dissolving 16.7% PLGA in ethyl acetate. Both solutions (polymer and drug) were mixed and transferred to 1% PVA solution to form oil-in-water (o/w) emulsions by vortex mixing or homogenization (3,400 rpm). The resulting emulsions were extracted with ethyl acetate to yield the porous microspheres, which were allowed to dry under vacuum at room temperature to remove any residual solvent. In contrast, the regular microspheres were prepared by dissolving polymer/drug (4:3) in methylene chloride which was homogenized in 1% polyvinyl alcohol solution to an o/w emulsion. Methylene chloride was removed by extraction that resulted in hardening of microspheres, which were further dried under vacuum. The dried microspheres were then washed with water and sieved through 25 microns and 212 microns sieves and lyophilized as powder cake. Chitkara and Kumar evaluated PLGA nanoparticles loaded with a highly hydrophilic drug, gemcitabine and coated with bovine serum albumin at the exterior layer.21 The nanoparticles with 243 nm in size and encapsulation efficiency was 40% and loading was 8.8%, showed a controlled release over 12 hours in vitro and cellular uptake within 2 hour in vivo.
These elements contribute to drug loading and release kinetics. Furthermore, the size of these microspheres typically ranged between 10-200 microns. With particle size 20 microns or lower, they are engulfed by immune cells; whereas, the large particles >200 microns cause inflammations. There are several techniques to produce these particles but the top down microfluidic method allows fabrication of microspheres with uniform size, shape and thickness, with higher encapsulation efficiency for hydrophilic and hydrophobic molecules.22
Preparation of PLGA Microspheres and Devices
There are several methods to prepare the PLGA nano/ microspheres. There are some tradition methods and others are more new technologies based. Those methods are illustrated in Figure 2 and their details of preparations are summarized below. Ruirui et al. describe some of these methods used for delivery of a number of drugs encapsulated in PLGA micros/nano-spheres.23

1. Emulsion and double emulsion solvent evaporation process (W/O/W) – this process is simple and requires the drug to dissolved in an aqueous solution (W) with or without excipients, and the PLGA is dissolved in organic solvents, preferably in methylene chloride or ethyl acetate (O). First W/O/ emulsions are formed by adding and mixing of aq. solution of drug in PLGA dissolved in organic solvent. The first emulsions (W/O) are then mixed with a second continuous aqueous solution containing a surfactant such as polyvinyl alcohol (PVA) to yield W/O/W emulsions. Following removal of organic solvent through diffusion or extraction or evaporation, it leads to solidification of the PLGA microspheres. The process limitations include solubility of drug in organic solvent used, and presence of residual solvents, and necessity for aseptic processing. Example of double emulsion process includes Lupron® Depot, see in Table2.
Table 1 Brain uptake of surface modified PLGA

2. Phase separation or coacervation method: This requires the preparation of first emulsions by addition of aq. drug into PLGA dissolved in methylene chloride. A coacervation agent, such as silicon oil is gradually added into primary emulsions to promote phase separation. As methylene chloride is extracted into the silicon oil, PLGA microspheres get precipitated, which are further hardened by washing with apolar solvents such as heptane or hexane. The process limitations include residual solvents, larger particles and necessity for aseptic processing. The approved drug Bydureon® is manufactured by this process, see Table 2. Arestin®,
as shown in Table 2, was also prepared by dissolving PLGA in dichloromethane and suspending the micronized minocycline hydrochloride in PLGA emulsion. Silicon oil was added as coacervate solvent and resulting dispersion was then solidified by discharging in hexane.24
3. Spray drying process: This atomization based process requires the spray drying of W/O emulsions prepared by aq. drug mixing with PLGA dissolved in methylene chloride. The resulting spray dried powder of PLGA microspheres entrapped with drug are dried to remove the residual solvents. The process limitations include thermal stress, residual solvents, and necessity for aseptic processing. The approved drug Supercure® MP is manufactured by this process and marketed in Japan, see Table 2. This is a rapid process, however, a low yield could be obtained due to adherence of the product along sidewall of drying chamber.
Download the full research article PLGA – A versatile copolymer for design and development of nanoparticles for drug delivery here (or click on the picture below)
Source: MedCrave, Journal of Analytical & Pharmaceutical research, PLGA – A versatile copolymer for design and development of nanoparticles for drug delivery, Volume 12 Issue 2 – 2023, Jim Huang, Shaukat Ali, Ascendia Pharma, Inc., USA, Correspondence: Shaukat Ali, Ascendia Pharma, Inc., 661 US, Highway One, North Brunswick, NJ 08902, Received: May 8, 2023 | Published: May 22, 2023
Visit our new Webinar:
Solving capping challenges using mannitol as an excipient model
Get more information & register here:
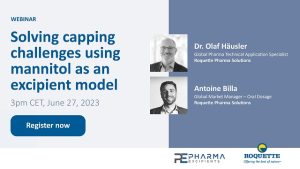