Recent Progress of Solid Lipid Nanoparticles and Nanostructured Lipid Carriers as Ocular Drug Delivery Platforms

Abstract
Sufficient ocular bioavailability is often considered a challenge by the researchers, due to the complex structure of the eye and its protective physiological mechanisms. In addition, the low viscosity of the eye drops and the resulting short ocular residence time further contribute to the observed low drug concentration at the target site. Therefore, various drug delivery platforms are being developed to enhance ocular bioavailability, provide controlled and sustained drug release, reduce the number of applications, and maximize therapy outcomes. Solid lipid nanoparticles (SLNs) and nanostructured lipid carriers (NLCs) exhibit all these benefits, in addition to being biocompatible, biodegradable, and susceptible to sterilization and scale-up. Furthermore, their successive surface modification contributes to prolonged ocular residence time (by adding cationic compounds), enhanced penetration, and improved performance. The review highlights the salient characteristics of SLNs and NLCs concerning ocular drug delivery, and updates the research progress in this area.
1. Introduction
Composition | Drug/Disease | Method of Preparation | Physicochemical Characteristics | Results | References |
---|---|---|---|---|---|
Tripalmitin Tween 80 Glycerol | Econazole/ Fungal keratitis | Microemulsion method | Size 19.05 ± 0.28 nm PDI 0.21 ± 0.01 ζ potential −2.20 ± 0.10 mV EE = 94.18 ± 1.86% | Slow and controlled drug release (within 96 h); Improved antifungal activity; Enhanced bioavailability—drug concentration was above MIC within 3 h after application. | [153] |
PrecirolATO 5 Pluronic F68 Stearyl amine | Natamycin/ Fungal keratitis | Hot emulsification-ultrasonication technique | Size 42 nm PDI 0.224 ζ potential 26 mV EE ≈ 85% | Prolonged drug release (within 8 h); Improved corneal penetration; Superior antifungal activity vs. free drug; Excellent ocular tolerability. | [154] |
Compritol 888 ATO Stearic acid Tween 80 Soy lecithin | Isoniazid/ Ocular tuberculosis | Microemulsion method | Size 149.2 ± 4.9 nm PDI 0.15 ± 0.02 ζ potential −0.35 ± 0.28 mV EE = 65.2 ± 2.2% | Prolonged drug release (48 h); Enhanced corneal permeability (1.6 fold); Improved ocular bioavailability (4.2 fold) vs. drug solution. | [155] |
Stearic acid Tween 80 Transcutol P | Clarithromycin/ Bacterial endophthalmitis | High-speed mixing and the ultrasonication method | Size 157 ± 42.4 nm PDI 0.13 ± 0.02 ζ potential −17.2 ± 3.1 mV EE = 81.3 ± 4.6 | Sustained drug release (~80% in 8 h); Improved transcorneal permeation and bioavailability compared to drug solution. | [156] |
Softisan 100 (Hydrogenated Coco-Glycerides) Suppocire NB (C10–C18 Triglycerides) Tween 80 Tegin O DOTAP DDAB | Sorafenib/ Uveal melanoma | Phase inversion temperature method | Size 127.85 ± 1.50 nm PDI 0.215 ± 0.014 ζ potential 20 mV EE= 75.0 ± 2.1% | Sustained drug release (less than 25% of encapsulated drug released after 72 h); Good physical stability, cytocompatibility and mucoadhesive properties of elaborated SLNs. | [157] |
Compritol 888ATO PEG 400 Poloxamer 188 Phospholipon 90H | Atorvastatin/ Age-related macular degeneration | Hot high-pressure homogenization | Size 256.3 ± 10.5 nm PDI 0.26 ± 0.02 ζ potential −2.65 mV EE= 73.1 ± 1.52% | Improved bioavailability (8-fold in aqueous humor and 12-fold in vitreous humor) vs. free drug; Proven safety in corneal/retinal cell lines; Successful delivery to the retina, confirmed by intact fluorescein-labeled SLNs. | [158] |
Compritol 888 ATO/Compritol HD5 ATO Pluronic F127 | Betulinic acid (BA) derivatives H3, H5 and H7/ Retinal diseases (diabetic retinopathy, age-related macular degeneration, choroidal neovascularization) | Microemulsion method | Size 58.5± 9.8 nm PDI 0.246 ζ potential 6.45 ± 5.58 mV EE = 75.10% | Improved drug delivery and enhanced anti-oxidative efficacy of BA derivatives; Suppressed glutamate-induced ROS production/necrosis in human Müller cells. | [159] |
Gelucire 44/14 Compritol ATO 888 Tween 80 | Etoposide/ Posterior segment-related diseases (e.g., age-related macular degeneration, diabetic retinopathy) | Melt- emulsification and ultrasonication technique | Size 239.43 ± 2.35 nm PDI 0.261 ± 0.001 EE 80.96 ± 2.21% | Sustained etoposide concentration of etoposide in vitreous body for 7 days after IV injection Better toxicological profile vs. etoposide solution. | [160] |
Stearic acid Sodium taurodeoxycholate Phosphati- dylcholine | Sutinib (Sb)/ Retinal diseases (age-related macular degeneration, diabetic retinopathy, retinal vein occlusions) | Microemulsion method | Size 140 nm PDI 0.20 | Excellent tolerability profile based on in vivo study on 20 albino rabbits; After IV injections, Sb SLNs didn’t cause any abnormalities in ocular morphology in contrast to polymeric nanocapsules. | [161] |
Chitosan Phospholipids (Lipoid S100) Glyceryl mono- stearate Tween 80 PEG 400 | Methazolamide/ Glaucoma | Emulsion-solvent evaporation method | Size 247.7 ± 17.3 nm PDI ζ potential 33.5 ± 3.9 mV EE = 58.5 ± 4.5% | Prolonged drug release compared to drug solution; Excellent tolerability and marked reduction in IOP vs. uncoated methazolamide SLNs. | [162] |
Compritol 888 ATO Pluronic F68 Tween 80 Glycerol | Δ9 -Tetrahydrocannabinol-valine-hemisuccinate/ Glaucoma | Ultrasonication | Size 287.80 ± 7.35 nm PDI 0.29 ± 0.01 EE = 93.57 ± 4.68% | Greater reduction in the IOP with respect to intensity and duration compared to pilocarpine/timolol maleate eye drops; High drug concentration in the iris/ciliary body and choroid/ retina. | [163] |
Legend: DDAB—Didodecyldimethylammonium bromide; DOTAP—Dioleoyl-trimethylammonium–propane chloride; EE—Entrapment efficiency; IOP—Intraocular pressure; MIC—Minimum inhibitory concentration; PDI—Polydispersity index; ROS—Reactive oxygen species.
Download the full article as PDF here: Recent Progress of Solid Lipid Nanoparticles and Nanostructured Lipid Carriers as Ocular Drug Delivery Platforms
or read it here
Gugleva, V.; Andonova, V. Recent Progress of Solid Lipid Nanoparticles and Nanostructured Lipid Carriers as Ocular Drug Delivery Platforms. Pharmaceuticals 2023, 16, 474.
https://doi.org/10.3390/ph16030474
Read more on Chitosan as a pharmaceutical excipient here:
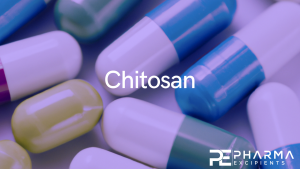