Inhalation Dosage Forms: A Focus on Dry Powder Inhalers and Their Advancements

Abstract
In this review, an extensive analysis of dry powder inhalers (DPIs) is offered, focusing on their characteristics, formulation, stability, and manufacturing. The advantages of pulmonary delivery were investigated, as well as the significance of the particle size in drug deposition. The preparation of DPI formulations was also comprehensively explored, including physico-chemical characterization of powders, powder processing techniques, and formulation considerations. In addition to manufacturing procedures, testing methods were also discussed, providing insights into the development and evaluation of DPI formulations. This review also explores the design basics and critical attributes specific to DPIs, highlighting the significance of their optimization to achieve an effective inhalation therapy. Additionally, the morphology and stability of 3 DPI capsules (Spiriva, Braltus, and Onbrez) were investigated, offering valuable insights into the properties of these formulations. Altogether, these findings contribute to a deeper understanding of DPIs and their development, performance, and optimization of inhalation dosage forms.
Introduction
Archives documented the use of inhalation therapy as a method of drug delivery back centuries, with historical records documenting the inhalation of various medicinal vapors and fumes for the treatment of respiratory diseases [1,2]. But it was not until recent decades that inhalation treatment made significant advancements, becoming a popular strategy for treating respiratory conditions like asthma and chronic obstructive pulmonary disease (COPD). Modern inhalation devices have contributed to this trend, with dry powder inhalers (DPIs) dominating the market because of their distinct benefits and potent drug delivery capabilities [3,4]. Inhalation therapy offers unique benefits in the treatment of respiratory disorders, primarily due to its targeted delivery approach. Inhalation ensures rapid drug absorption and deposition at the site of action by directly delivering medications to the lungs [2,5], therefore minimizing systemic exposure and potential systemic side effects, and thus optimizing and improving therapeutic outcomes, patient compliance, and overall quality of life. Among the diverse range of inhalation devices available, DPIs have become an essential choice, attracting considerable interest.
The popularity of these devices is due to a number of features that solve the drawbacks and limitations of conventional inhalers, namely nebulizers and metered-dose inhalers (MDIs). DPIs are renowned for their propellant-free formulation, eliminating concerns over environmental impact and propellant-related adverse effects [4,6,7]. Instead, DPIs employ dry powder formulations, which offer an increased chemical stability compared to their liquid-based counterparts [8]. Furthermore, DPIs offer ease of use and require minimal patient coordination during administration, rendering them accessible and suitable for patients of all ages, including children and the elderly. In addition to being breath-actuated, DPIs also do not require the use of a spacer [7,9]. Clear indications of the growing interest in DPI research were obtained from a comprehensive search of the clinicaltrials.gov database, which revealed a notable rise in clinical studies centered around DPIs. Among these clinical trials, 4 are awaiting participant recruitment, while 15 are actively recruiting. Additionally, 1 study is listed as active but not recruiting, 395 have successfully concluded, and 15 were terminated [10].
The success of DPIs, however, hinges on certain aspects, including the physicochemical properties of the drug formulation (moisture sensitiveness), the design and functionality of the inhaler device, and the patient’s inhalation technique [9,11]. Achieving consistent drug delivery in the lungs remains a challenge in DPI development, necessitating ongoing research and innovation to optimize aerosol generation and particle dispersion upon inhalation. Advances in device design and formulation development have played a pivotal role in overcoming these challenges, elevating DPIs to new heights of efficiency and therapeutic performance.
This review aims to offer a comprehensive examination of inhalation therapy, with a primary focus on DPIs and their associated formulations. The scope of this review encompasses the various categories of DPIs available, including single-dose and multi-dose reservoir DPIs, each with distinct powder dispersion mechanisms. Moreover, we will explore the fundamental attributes of DPI design, considering factors such as user-friendliness, dose accuracy, portability, and hygienic considerations. Furthermore, this review will explore the crucial role of formulation technology in DPI development, addressing strategies to optimize drug stability, enhance fine particle fraction (FPF), and achieve consistent dose uniformity. Additionally, we will explore the increasing adoption of DPIs for delivering biotech drugs, as well as the incorporation of lipid-based or polymer-based carriers to enhance drug bioavailability and therapeutic efficacy. By examining the versatile nature of DPI formulation and delivery devices, this review aims to provide valuable insights to the ongoing efforts in optimizing inhalation therapy and ultimately enhancing patient outcomes.
3.2. Excipients
Excipients offer the possibility to improve the non-pharmacologic properties of a formulation. They were formerly deemed to be inert substances but have since proved that they are, on the contrary, useful substances which can be designed to improve the formulation by decreasing the particles adhesion and ameliorate powder dispersion. They can reinforce the physical or chemical stability of a formulation, its mechanical properties, improve the absorption or release of the API, or even act as disintegrant, binders, lubricants, filler agents (as mentioned earlier in case of a small drug content), sweeteners, and coloring/identification agents [32,50].
As discussed previously, in terms of pulmonary drug delivery, excipients are specifically required in the formulation to reach the best possible size, thus they are typically found in relatively high amounts in contrast to the API. They supply a bulk mass, hence ameliorating the handling, metering, and dispensing of the drug. The excipient particles are usually produced through milling [32]. Their use may also improve the patient’s compliance, as it can enhance the taste but most importantly the sensation felt by the patient upon inhalation, therefore giving feedback to the patient that the dose was indeed administered [51].
Several components with the potential to improve the pulmonary delivery could also irritate the lungs. Consequently, the excipient options are limited to those easily metabolized or cleared. Among these excipients, lactose (α-lactose monohydrate) is the most frequently used. It has a lengthy use as an excipient in oral formulations and is now present in more than ¾ of the most common marketed DPIs [52,53]. This is due to its multiple advantageous properties such as [54,55]:
- Physico-chemical stability and compatibility with most low molecular weight drugs;
- Safe toxicological profile;
- Availability and affordability;
- Less hygroscopic than other sugars.
Its highly crystalline nature and good flow properties make it a preferred carrier for APIs in DPIs [55]. However, lactose is unsuitable for diabetic or lactose-intolerant patients since it eventually gets swallowed following its impact on the oropharynx. It can pose risks for individuals with cow’s milk protein allergy (CMPA), as it may contain allergenic milk proteins. Inhalation of these milk proteins may potentially trigger severe allergic reactions [56], and in rare cases, even lead to fatal outcomes [57]. Several instances of lactose in DPIs being contaminated with milk proteins led to allergic reactions, mostly in children (aged 6 to 10) [58,59,60,61] and an adult woman [62]. Lactose is present in 5 (all of which are DPIs) of the 17 inhaled asthma medicines registered by the Food and Drug Administration (FDA). Although anaphylactic reactions from lactose-containing DPIs are mentioned in package inserts, the incidence of such reactions is unknown [60].
Furthermore, a recent study investigated the use of DPIs containing lactose in patients with CMPA. Out of 77 doctors who responded, 45.5% were unaware that DPI leaflets listed CMPA as a contraindication to DPI administration. Additionally, almost all participants were not aware of any systemic allergic reactions in CMPA patients who received lactose-containing DPIs, least of all anaphylactic reactions [63]. Moreover, low-dose APIs in lactose-based adhesive mixtures delivered by a DPI with no clear efficient dispersion principle is a drawback in dry powder inhalation. Despite the addition of magnesium stearate, these mixtures only produce FPFs of up to 40 to 50% of the label claim. The average value among all marketed DPIs today is about 30%, indicating that there is still potential for progress and improvement [64]. Due to these reasons, mannitol, for instance, could represent an alternative to lactose [55,64,65]. Various alternative carrier materials, mainly sugars, have been investigated, leading to varying results depending on the type of inhaler used or even the type of API present in the formulation used. Furthermore, many of the carrier materials that have been tested are not yet authorized for inhalation by the FDA. Another cause for the substitution of lactose in the future is the growing interest in using it as well as lactic acid for other purposes: lactose is sought-after as a sweetening, stabilizing, and moisture-retaining component in food items. Meanwhile, lactic acid is employed as an acidifier in fruit juices and beverages, a preservative, and a flavoring ingredient in pharmaceutical and cosmetic products [64].
It is essential to avoid reducing sugars in formulations comprising amino-group-containing APIs to prevent Maillard reactions and instability [55,66]. To overcome this, non-reducing polysaccharides and non-reducing disaccharides and other sugars are being considered as carriers [54]. Furthermore, a combination of fine carrier particles with the API at an equivalent size range has proven to be a significant factor in the improvement of the formulation performance. Fine lactose particles occupy possible API binding sites on the coarser lactose particles, thus reducing the drug-carrier interactions, as seen with salbutamol sulfate, for example [67]. This improvement is associated with the presence of active sites on the carrier’s surface [67,68,69]. In conclusion, while lactose is common, it may not always be the ideal excipient due to its drawbacks, leading to the development of alternatives such as FDA-approved Mannitol for inhalation use.
Another possibility is to switch from a passive DPI to an active one, achieving dispersion through the use of external energy, or even to exclude the use of a carrier altogether. It is important to note that excipients are not always necessary in a DPI formulation, examples of that are the Oxis Turbohaler® (formoterol) or the Pulmicort Turbohaler® (budesonide).
Download the full article as PDF here: Inhalation Dosage Forms
or read it here
Magramane, S.; Vlahović, K.; Gordon, P.; Kállai-Szabó, N.; Zelkó, R.; Antal, I.; Farkas, D. Inhalation Dosage Forms: A Focus on Dry Powder Inhalers and Their Advancements. Pharmaceuticals 2023, 16, 1658. https://doi.org/10.3390/ph16121658
Watch our recorded free webinar “Spray drying with Shin-Etsu, ProCepT and Xedev” here:
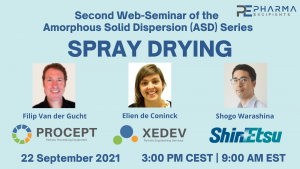