Enhancement of inhaled micronized powder flow properties for accurate capsules filling
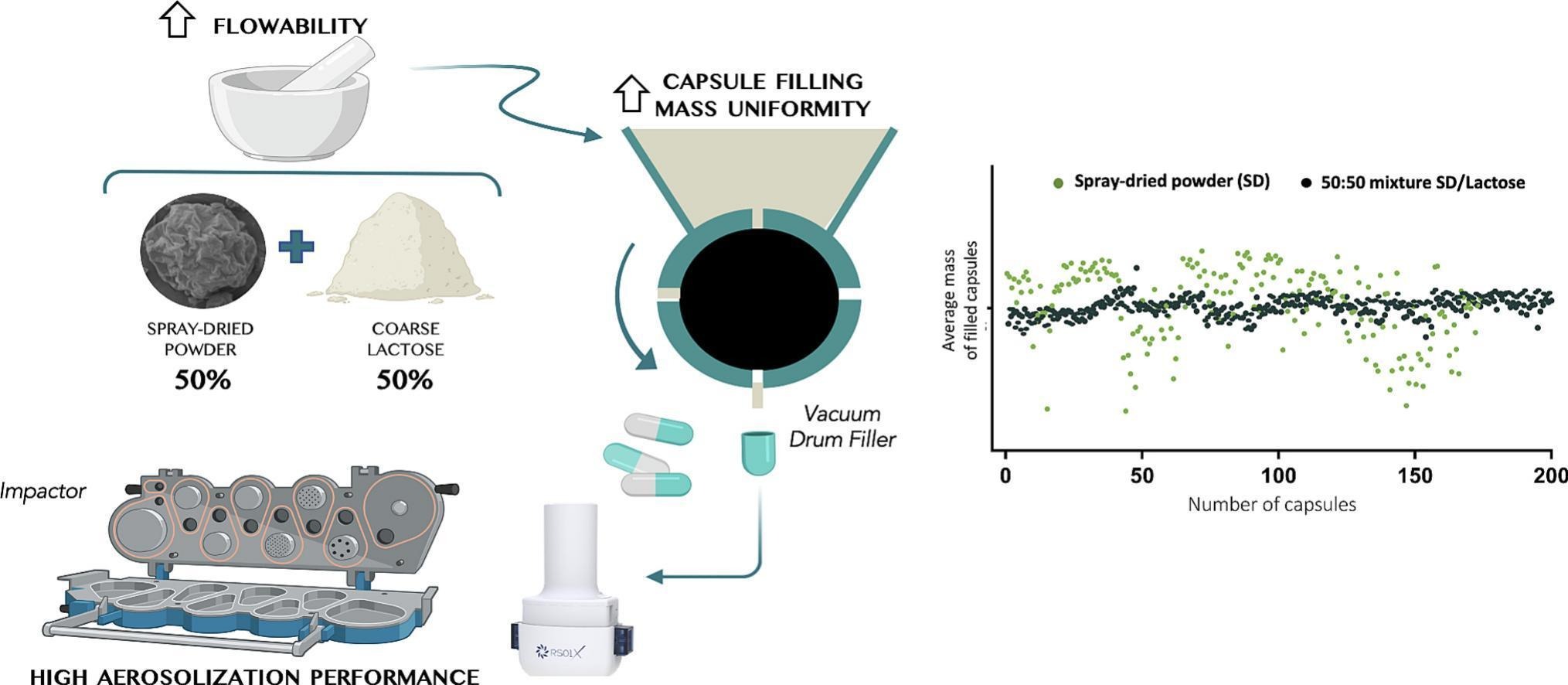
Introduction
Dry powder inhalers (DPI) are gaining popularity in the field of pulmonary treatments due to their numerous advantages. Compared to pressurized metered-dose inhalers (pMDI), soft-mist inhalers (SMI), and nebulizers, which utilize liquid formulations, DPIs employ solid-state formulations, thereby conferring increased drugs and microbial stability [1,2]. Furthermore, DPIs offer several benefits, including propellant-free operation, elimination of the need for hand-mouth coordination and the ability to deliver high doses of drug, making them an appealing option for pulmonary drug administration [3,4]. To achieve therapeutic efficacy, particles or droplets must have an aerodynamic diameter within the range of 1–5 μm, enabling deep lung deposition while minimizing impaction in the upper respiratory tract or diffusion [5,6]. However, concerning specifically powders, the requirement for small particle size leads to interparticulate interactions characterized by cohesive and adhesive forces, such as van der Waals or electrostatic forces, resulting in reduced powder flowability [7]. Apart from particle size, powder flow properties are influenced by other particle characteristics, including shape, surface roughness, and size distribution, all of which contribute to the handling challenges and can pose limitations in industrial processes such as capsules or container filling [8,9].
Highlights
- Optimizing inhalation powder flowability enhances industrial processability.
- Blend of spray-dried powder and coarse lactose lead to homogeneous ordered mixture.
- Mixtures do not reduce aerosolization performance of engineered powder.
- Mixtures greatly improve capsule filling mass uniformity (vacuum capsule filler).
To enhance the bulk properties of powder formulations used in DPIs, a common approach involves combining micronized active pharmaceutical ingredient (API) with a carrier, typically a coarse particle ranging in size from 50 to 200 μm, commonly lactose monohydrate [10]. Generally, >90% of the mixture consists of the carrier. This results in an ordered mixture, also known as an adhesive blend, which consists of a mixture of micronized particles and significantly larger particles (>100 μm) [5,11,12]. Within the blend, adhesive forces facilitate interactions between API particles and the carrier surface, resulting in their attachment [13]. For effective deposition in the deep lung, micronized particles must detach from coarse particles [12,14]. Achieving a balance between formulation stability and uniformity through interactions and ensuring adequate separation of the API from the carrier is essential to obtain a high lung deposition [12,13,15]. The dissociation process is influenced by factors such as the formulation itself, including particle size distribution and particle interactions. The deagglomeration of the powder also depends on external factors, such as the type of inhaler used and its resistance and the patient’s inspiratory flow rate [2,16]. During inspiration, the separation of the mixture results in carrier impaction in the upper respiratory tract, making API particles available for deposition to the target site [10]. However, the interaction forces between the carrier and APIs are complex, and developing inhalation powders with high pulmonary deposition remains a significant challenge, even with the addition of fines. Indeed, currently available DPIs typically achieve a fine particle fraction (<5 μm) of approximately 20–30%, resulting in a considerable loss of API in the throat-mouth region and within the device [15,17,18].
In recent decades, extensive research has been conducted on particle engineering in the context of developing inhalation powders, including both carrier-based and carrier-free formulations. Particle engineering allows the design of particles with desirable properties to achieve optimal pulmonary deposition [19]. Various particle properties, such as size, density, shape, drug stability, surface properties, crystallinity and hygroscopicity, significantly impact powder aerosolization [3,20]. Among the diverse methods available for developing such complex particles, spray-drying is currently the most commonly employed approach [21]. Spray-drying is a pharmaceutical manufacturing process wherein solution, emulsion or suspension containing the desired API is atomized into fine droplets and subsequently dried using hot gas, resulting in the formation of dry particles [22]. This method is frequently used due to the ease of modification of various parameters, such as atomization and drying conditions, liquid formulation, and equipment design [13,23]. Spray-drying allows the precise control of the attributes of the particles, enabling modification of several properties, thereby enhancing the aerosolization performance of the resulting powder [13].
Particle engineering involves the production of a wide range of particle types, including Pulmosphere® and deflated particles. The Pulmosphere® process, employed in the production of TOBI® Podhaler, a commercially available DPI, involves the generation of porous low-density particles through emulsion atomization [22]. The distinct porous morphology and hydrophobic surface result in the development of a carrier-free formulation that exhibits reduced interparticle cohesive forces [24]. Consequently, this formulation demonstrates enhanced aerodynamic performance, ultimately improving the overall efficiency of the powder [25].
Besides investigating porous particle shapes, deflated morphologies are also under investigation. Cui et al. [26] have synthesized Netilmicin-based particles with a corrugated surface, while Mangal et al. [27] have investigated the effect of L-Leucine concentration on the deflated morphology of the particles. Dufour et al. [28] successfully developed deflated particles by spray-drying a solution containing hydroxypropyl-β-cyclodextrin (HPB) and budesonide [28]. Impaction tests revealed significantly superior aerosolization performance compared to Miflonide®, a carrier-based formulation [28]. In addition, Lechanteur et al. [29] demonstrated the impact of the composition of the spray-dried liquid feedstock on the morphology of the particles. The authors defined a new deflation ratio as the product of the number of dimples and their depth, which exhibited a discernible correlation with the aerosolization performance of the resulting powder [29]. Subsequently, employing a Design of Experiment methodology, the intricate relationship between specific spray-drying parameters and powder performance was explored [30]. These analyses led to the identification of optimal powder formulation and drying parameters in our group.
This formulation comprises two carbohydrates (HPB, 50% m/v; raffinose, 50% m/v), an amino acid (L-leucine, 5% m/v), and two drugs (budesonide, 0.66% m/v; formoterol fumarate, 0.02% m/v). HPβCD was chosen for its ability to produce particles with desirable aerodynamic properties, facilitating effective aerosolization. The addition of raffinose aimed to optimize the formulation’s cost-effectiveness, while leucine was included to provide moisture protection. Consequently, the optimized powder demonstrates a deflated morphology and exceptional aerosolization performance, achieving a fine particle fraction of nearly 60% for both drugs. These results hold significant promise in the context of inhaled powder development. However, the suboptimal flow characteristics resulting from the small particle size (d0.5 = 2.56 μm) underscore the need to address this challenge, as it is crucial for enhancing the flowability of the spray-dried (SD) powder and enabling reproducible and accurate capsule filling. Conventional methods such as dry and wet granulation are impractical for inhalation powders [31]. Furthermore, the process of creating soft pellets remains poorly understood from a physical standpoint, leading to inadequate control [32]. For these reasons, this research aims to explore an alternative option, which involves combining micronized atomized powder with larger lactose particles. Two different types of lactose, namely Inhalac® 70 and Inhalac® 230, were used in order to evaluate their size and surface properties (roughness, area…) on blend homogeneity, blend flowability, and aerosolization performance. Five adhesive mixtures with varying lactose proportions, ranging from 0% to 100%, were prepared and studied. Moreover, a capsule drum filler was employed to assess the reproducibility of filling, considering an industrial perspective. Finally, the dissociation of the powder during inhalation was investigated using different inhaler resistances.
Overall, this study aims to improve the flow characteristics of the micronized powder, enhancing processability while preserving the superior performance achieved through particle engineering. The findings presented herein contribute valuable insights to address critical issues in the pharmaceutical manufacturing of inhalation powders.
Read more here
Materials
Budesonide (BUD) micronized and formoterol fumarate dihydrate (FOR) were used as model APIs and were supplied from Minakem (Dunkerque, France) and CHEMO Industriale chimica (Saronno, Italy), respectively. Hydroxypropyl-β-cyclodextrin (HPB) was kindly provided by Roquette (Lestrem, France). Raffinose pentahydrate (RAF) was obtained from Acros Organics (ThermoFisher Scientific, Geel, Belgium). L-Leucine (LEU) was purchased from Tokyo Chemical Industry (Tokyo, Japan). Inhalac® 70 (Lac70) and Inhalac® 230 were obtained by Meggle.
Eva Gresse, Justine Rousseau, Myriam Akdim, Audrey Du Bois, Anna Lechanteur, Brigitte Evrard, Enhancement of inhaled micronized powder flow properties for accurate capsules filling, Powder Technology, 2024, 119576, ISSN 0032-5910, https://doi.org/10.1016/j.powtec.2024.119576.
Read more interessting articles on Dry powder inhalers (DPI) here:
- Inhaled dry powder liposomal azithromycin for treatment of chronic lower respiratory tract infection
- A Novel Combined Dry Powder Inhaler Comprising Nanosized Ketoprofen-Embedded Mannitol-Coated Microparticles for Pulmonary Inflammations
- Improving Inhalation Performance with Particle Agglomeration via Combining Mechanical Dry Coating and Ultrasonic Vibration
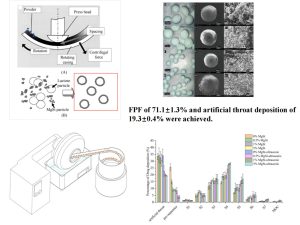