Excipients in Pharmaceutical Additive Manufacturing: A Comprehensive Exploration of Polymeric Material Selection for Enhanced 3D Printing
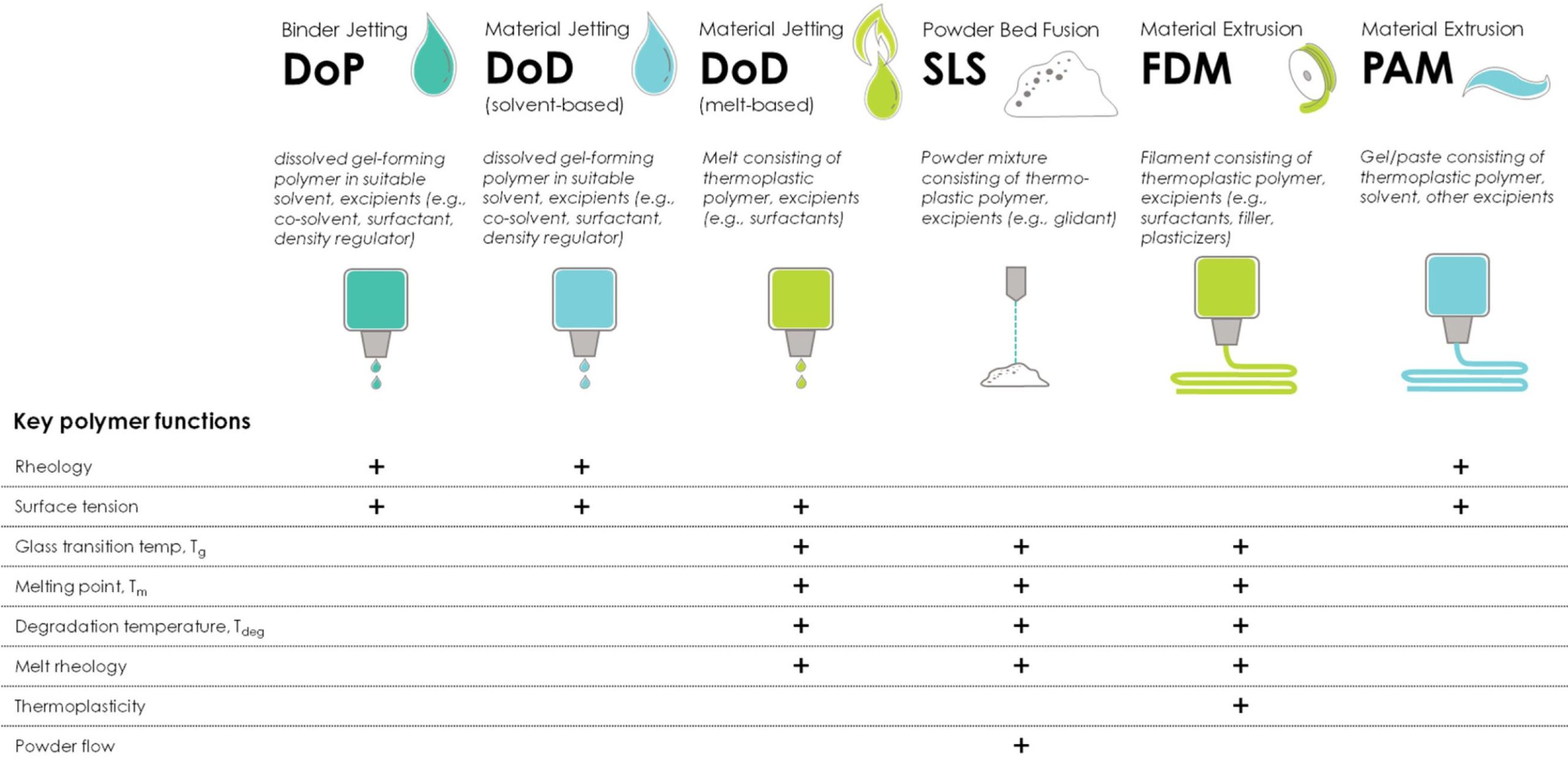
Abstract
This review provides a comprehensive overview of additive manufacturing (AM) or 3D-printing (3DP) applications in the pharmaceutical industry, with a particular focus on the critical role of polymer selection. By providing insights into how material properties influence the 3DP process and the quality of the final product, this review aims to contribute to a better understanding of the interplay between polymers and pharmaceutical 3DP. As 3DP technologies are increasingly integrated into pharmaceutical sciences, this review contributes insights into the nuanced process of polymer selection, serving mainly as a foundational guide for researchers and formulators new to the subject seeking to harness the full potential of pharmaceutical 3DP by understanding the physicochemical properties, roles, and functions of used polymers in 3D-printed dosage forms and medical devices.
Introduction
In recent years, additive manufacturing (AM), popularly known as three-dimensional printing (3DP), has been widely applied to the fabrication of prototypes through to functional parts in various manufacturing areas, including the pharmaceutical industry. The term “3D printing” technically refers to a broad collection of additive technologies based on the deposition of materials, like creating objects from the bottom up or layer by layer. Despite the technical differentiation between the terms, “3D printing” has become the common term, and thus, 3DP and AM are used interchangeably in this review to refer to the collection of AM technologies that will be discussed.
Although there is a growing demand for technologies that enable pharmaceutical manufacturers to offer personalized and customized treatments, the uptake and utilization of 3D printing in healthcare applications is relatively new. To date, this has predominantly involved the printing of plastics and metals, such as surgical planning, prosthetics, or reconstructive surgery, and has been used to create tailored bone inserts for use in complex reconstructive surgery [1,2,3]. On the contrary, the 3D printing of pharmaceuticals is less advanced. For example, in 2015, the US regulatory agency the Food and Drug Administration (FDA) approved the first 3D-printed pill, Spritam®, from Aprecia Pharmaceuticals (Blue Ash, OH, USA) [4]. The applied AM process enabled the manufacturers to produce orally disintegrating tablets with a higher drug load, compared to conventional levetiracetam solid-dosage forms, and a short disintegration time due to the porous structure of the printed tablets. Like any other solid oral dosage form, the FDA affirmed that the 3D-printed tablet must comply with existing manufacturing and control regulations such as 21 CFR 200 and 21 CFR 300 [5], and they are approved according to existing regulatory and quality frameworks [6].
In 2016, the FDA released new draft guidance on the Technical Considerations for Additive Manufactured Devices to advise manufacturers who are producing devices through 3D-printing techniques. With the official document issued in 2017, the guidance provides manufacturers with recommendations for two major areas of medical device development: device design and manufacturing, and device testing [7]. Specifically for device testing, which includes device characterization, validation, and verification, the FDA recognized the unique challenges associated with AM. This starts with the impact of the 3D printer and post-printing processes on the final device performance; however, it also includes the importance of material control and the need for a robust process validation and acceptance protocol. Since then, there has been an increased interest in 3DP technologies across the pharmaceutical industry.
The main advantages associated with the use of AM technologies in the pharmaceutical area include customization, i.e., the development of personalized medications such as individualized printed dosage forms and implants [8]. Furthermore, AM technologies can contribute to bringing new therapies to the market via small-scale production in a brief time [9]. This is beneficial for the development of new drugs and therapies, especially when utilized for parenteral applications. Finally, AM helps to advance the personalization of medicines by enabling fabrication according to the patient’s treatment and dosage needs, e.g., for geriatric and pediatric patient populations who lack suitable available formulations [10], and blind or visually impaired individuals [11].
During the development of AM technologies, various definitions and terms were used that referenced specific application areas and even trademarks. The standard ISO/ASTM 52900:2021 [12] was created as a comprehensive framework to facilitate and widen communication and innovation between people involved in AM technologies. Consequently, it plays an important role in the pharmaceutical/medical sector by providing a standardized approach. According to ISO/ASTM 52900:2021, there are seven different process categories in additive manufacturing [12]. These are:
- Binder jetting;
- Material jetting;
- Powder-bed fusion;
- Material extrusion;
- VAT photopolymerization;
- Sheet lamination;
- Directed energy deposition.
The first five AM technologies from the list above have been used for pharmaceutical applications. Detailed overviews and descriptions referring to these AM technologies are widely available, and thus, dedicated reviews are recommended to avoid replicating that information [13,14,15,16,17,18,19]. The same applies to characterization techniques, ranging from polymer screening, process monitoring, and optimization to intermediate and final product attributes of the printed drug products. Consequently, the reader is referred to available reviews [20]. Rather, this review should provide some guidance and direction on how to choose polymeric excipients based on their physicochemical material attributes and their influence on printability. Nevertheless, selecting a polymer with suitable feedability, printability, and product characteristics is not an easy task and requires a good understanding of how the characteristic properties of utilized polymeric excipients influence the printing process, and ultimately, final product quality.
Polymers (alone or together with other pharmaceutical excipients) play an important role in the manufacturing of conventional or advanced drug-delivery systems. In conventional manufacturing technologies, they are generally used as binders, fillers, lubricants, and solubility enhancers in oral solid dosage forms such as tablets and capsules, as well as emulsifying, suspending, or stabilizing agents and rheology enhancers in liquid and semisolid preparations. Polymers are macromolecules consisting of repeating units (or monomers) throughout their chains [21]. When two, three, or four monomer units are attached to each other, the product is known as a dimer, trimer, or tetramer, respectively. Products containing more than 200 monomer units are simply called polymers.
Polymers can be classified in different ways [21] based on the following:
- Their origin (naturally occurring, semi-synthetically modified, or synthetically manufactured), e.g., while cellulose is the basic structural component of plant cell walls and thus the most abundant of all naturally occurring polymers, cellulose ethers such as hydroxypropyl cellulose (HPC) or hydroxyethyl cellulose (HEC) are semi-synthetic polymers that become water-soluble due to the introduction of their side chains. On the other hand, polymers such as polyvinylpyrrolidone (PVP) and copovidone (PVPVA) are synthetic polymers obtained by the radical polymerization of their monomers.
- Types of monomer(s) (homopolymer or copolymer), e.g., PVP is obtained from the polymerization of N-vinyl-pyrrolidone (NVP) solely, while PVPVA is a copolymer obtained from the polymerization of NVP with vinyl acetate.
- Gross topology of the chain structure (linear, branched, or crosslinked), e.g., whether the polymer chain or chain assembly can be mapped onto a one- (linear), two- (branched), or three- (crosslinked network) dimensional object.
- Interaction with water (water-soluble or water-insoluble), e.g., hydroxypropyl cellulose (HPC) is a water-soluble cellulose ether, while ethyl cellulose (EC) does not dissolve in water.
Since all these properties will impact the final properties of the material [22], the right selection of polymers is crucial to facilitate the processing of the active pharmaceutical ingredient (API) into a dosage form, e.g., by providing the required physical stability to incorporated APIs or to modulate the drug dissolution and release. Thus, it is important to understand which polymeric excipients are suitable for which 3D-printing process, since polymer selection based on printing properties is key for the successful printing of the final drug product. Furthermore, despite being a processing aid in the printing process, the material choice will impact the mechanical properties and drug release and, thus, the overall performance of the final printed product.
This review is structured into three parts that should help readers in understanding the complexities of polymer printability in AM:
- Explanation of the significance of material properties in successful 3D printing
- Introduction to the key process stages of 3D printing—feeding, deposition and adhesion—and a discussion about the importance of these key determinants of printability.
- Discussion of pharmaceutical polymers (cellulose ethers, polyvinyl polymers, and bioresorbable polymers) and their material properties (solubility, viscosity, rheology, and mechanical characteristics) for their use in different AM technologies.
The selection and properties of materials are directly linked to the AM process, and the working principle of each technique defines the required properties of the material to be used. Table 1 lists the commonly used polymers for pharmaceutical AM applications. While some polymers such as ethyl cellulose, hydroxypropyl methylcellulose, and povidone (PVP) are added to the printing ink due to their rheological properties or impact on the ink’s surface tension [23], other polymers may cause nozzle clogging when added to the printing ink and, therefore, might be preferably included in the solid substrate [24]. The same polymers are used in PAM extrusion technologies; however, in this case, they are added to help wet the dry solid substrate, thus contributing to interlayer adhesion, and consequently, they support the mechanical structure of the final printed product [25]. In recent years, thermoplastic polymers such as copovidone and povidone, as well as cellulosics such as ethyl cellulose and hydroxypropyl cellulose, have been extensively used in spray-drying and hot-melt extrusion applications to solubilize poorly soluble active pharmaceutical ingredients (APIs).
In addition to solubilization, the same polymers also help stabilize the metastable amorphous form of the API, leading to the formation of amorphous solid dispersions [26]. Accordingly, these polymers have been used in melt-based AM technologies such as fused deposition modeling (FDM) as well; however, not all the thermoplastic polymers traditionally used in hot-melt extrusion (HME) perform well in FDM. The necessary higher processing temperatures in FDM compared to HME, as well as the requirements to show immediate drug release, might limit the use of some HME polymers in FDM [27]. Table 1 provides a summary of the commonly used polymers utilized in pharmaceutical-relevant 3D-printing applications. Publications selected for this review had to fulfill the following criteria:
Table 1. Commonly used polymers for pharmaceutical AM applications. DoP = drop-on-powder printing; DoD = drop-on-demand printing; SLS = selective laser sintering; FDM = fused deposition modeling; PAM = pressure-assisted micro-syringe.
Additive Manufacturing Technology | |||||
---|---|---|---|---|---|
Binder Jetting | Material Jetting | Powder-Bed Fusion | Material Extrusion | Material Extrusion | |
Polymer (Alphabetical Order) | DoP | DoD | SLS | FDM | PAM |
Carboxymethylcellulose sodium (Na-CMC) | [28,29,30] | ||||
Copovidone (PVP/VA) | [31,32] | [11,33,34,35, 36,37] | [9,38,39,40,41, 42,43,44,45,46] | ||
Ethyl cellulose (EC) | [47,48,49] | [50,51] | [38,39,52,53, 54,55,56] | ||
Hydroxyethyl cellulose (HEC) | [57,58] | [59,60,61] | |||
Hydroxypropyl cellulose (HPC) | [24] | [39,40,43,46, 52,53,55,57, 62,63, 64,65] | [66,67] | ||
Hypromellose (HPMC) | [47,48,49] | [33,68] | [38,42,52,53,69] | [25,70,71,72, 73,74,75,76] |
|
Polyglycolic acid (PGA) | [77,78] | ||||
Polycaprolactone (PCL) | [79] | [77,80,81,82, 83] | [84] | ||
Polylactic acid (PLA) | [85] | [77,78] | |||
Poly(lactic-co-glycolic) acid (PLGA) | [86,87] | [86,88] | |||
Poly(L-lactide-co-ε-caprolactone) (PLCL) | [89] | [90] | [91] | ||
Povidone (PVP) | [47,48,49,92, 93,94,95,96, 97,98,99,100] | [23] | [41,80,101,102, 103] | [28,71,72,73, 75,76,104,105, 106] |
- The additive manufacturing technology was clearly described and fit into the five ISO/ASTM 52900:2021 pharmaceutically relevant categories listed above.
- The polymers described were relevant for the functionality of the printed drug product and the authors provided sufficient information on the polymer types and grades used.
- The scope of the work was pharmaceutical or biomedical research with a particular focus on processability.
- There was no restriction on the publication period for manuscripts; however, more focus was put on papers published within the last 5 years.
Download the full article as PDF here: Excipients in Pharmaceutical Additive Manufacturing
or read it here
Muehlenfeld, C.; Duffy, P.; Yang, F.; Zermeño Pérez, D.; El-Saleh, F.; Durig, T. Excipients in Pharmaceutical Additive Manufacturing: A Comprehensive Exploration of Polymeric Material Selection for Enhanced 3D Printing. Pharmaceutics 2024, 16, 317. https://doi.org/10.3390/pharmaceutics16030317
Read more on 3D Printing here:
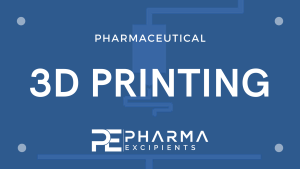