Spray-Congealing and Wet-Sieving as Alternative Processes for Engineering of Inhalation Carrier Particles: Comparison of Surface Properties, Blending and In Vitro Performance
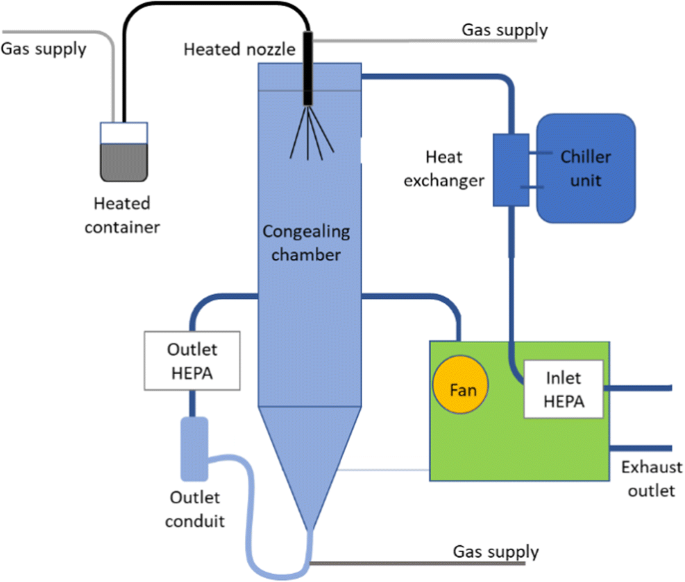
Purpose
Traditionally, α-lactose monohydrate is the carrier of choice in dry powder inhaler (DPI) formulations. Nonetheless, other sugars, such as D-mannitol, have emerged as potential alternatives. Herein, we explored different particle engineering processes to produce D-mannitol carriers for inhaled delivery.
Methods
Wet-sieving and spray-congealing were employed as innovative techniques to evaluate the impact of engineering on the particle properties of D-mannitol. To that end, the resulting powders were characterized concerning their solid-state, micromeritics and flowability. Afterwards, the engineered carrier particles were blended with inhalable size beclomethasone dipropionate to form low dose (1 wt%) DPI formulations. The in vitro aerosolization performance was evaluated using the NEXThaler®, a reservoir multi-dose device.
Results
Wet-sieving generated D-mannitol particles with a narrow particle size distribution and spray-congealing free-flowing spherical particles. The more uniform pumice particles with deep voids and clefts of wet-sieved D-mannitol (Pearl300_WS) were beneficial to drug aerosolization, only when used in combination with a ternary agent (10 wt% of ‘Preblend’). When compared to the starting material, the spray-congealed D-mannitol has shown to be promising in terms of the relative increase of the fine particle fraction of the drug (around 100%), when used without the addition of ternary agents.
Conclusions
The wet-sieving process and the related aerosolization performance are strongly dependent on the topography and structure of the starting material. Spray-congealing, has shown to be a potential process for generating smooth spherical particles of D-mannitol that enhance the in vitro aerosolization performance in binary blends of the carrier with a low drug dose.
Download the full article as a PDF here or read it here
Materials: D-mannitol (Pearlitol® 300 DC) was purchased from Roquette (France) and α-LH (CapsuLac® 60 and InhaLac® 120) was obtained from Meggle (Germany). The model API, beclomethasone dipropionate (BDP) with a mean particle size of 1.05 μm was provided by Chiesi Pharmaceutici S.p.A, Italy. Magnesium Stearate (MgSt) as well as acetone were purchased from Merck KGaA, Germany.
Introduction
In dry powder inhalation (DPI), drugs are delivered as solid particles to the lung. In order to reach the lower respiratory airways, the drug particles have to be in the size range of 0.5/1.0 to 5.0 μm (1). Due to their very large surface to volume ratio, the particles in the aforementioned size range, are cohesive and possess poor powder flow. The flowability and dispersion of the inhaled active pharmaceutical ingredient (API) particles can be improved by specifically tailoring their properties (e.g., to free-flowing large porous particles) or blending with excipients. Traditionally, the drug particles are blended with coarse excipient ones (Dv0.5 ≥ 20 μm) in a practice known as adhesive mixing. Adhesive mixing aims to attach the smaller API particles onto the surface of larger excipient ones. Likewise, the excipient functions as a ‘carrier’ and such formulations are called carrier-based DPI formulations.
α-lactose monohydrate (α-LH) particles are typical DPI carriers presenting a tomahawk shape and a mean particle size (Dv0.5) between 50 μm and 250 μm, depending on the device used. α-LH can be blended solely with the API, forming binary blends or can be mixed with the drug and other excipient particles that are added in order to improve the aerosolization. These excipient particles, presenting a size smaller than the coarse carrier, are usually known as fines. Excipient fines are usually magnesium stearate or lactose particles alone or in a mixture. Fine particles are known to aid the performance of DPIs by a variety of mechanisms (2,3,4). Although α-LH has been typically used as a carrier, its complex solid state, encompassing 2 anomeric forms, 2 anhydrous polymorphs and 1 hydrate of its α-anomer as well as an amorphous phase makes engineering of lactose particles quite challenging. Moreover, high temperature engineering processes cannot be applied to lactose due to its degradation upon melting. Thus, D-mannitol, a sugar approved for inhalation (5), is regarded as a good alternative to lactose. D-mannitol crystals exist as two polymorphs (the α- and β-forms) with very similar thermodynamic behavior that are monotropically related, and a metastable form enantiotropically (δ-form) related to the β-polymorph. Although, an amorphous phase and a hemi-hydrate of the β-polymorph are known, these forms cannot be easily obtained under ambient conditions. Moreover, D-mannitol does not degrade upon melting offering the possibility to process it at high temperatures. Thus, D-mannitol has been extensively studied as a potential carrier for DPIs in recent years (6,7,8,9). In 2017, Merck has started commercializing a grade of D-mannitol intended to be used as a carrier in DPI formulations (10).
During inhalation, carrier particles will impact the upper respiratory tract and get swallowed or expectorated. Thus, although drug particles need to attach with sufficient force to the carrier surface to be properly handled during manufacturing, and in particular to facilitate dose metering, the attachment also has to be loose enough to allow drug detachment during inhalation. DPI formulations are commonly delivered using inhalation devices that rely on the inspiratory forces of patients to fluidize the powder and promote detachment. Hence, when designing excipient particles for inhalation a combination of factors related to the carrier and API combination as well as type of inhalation device and its dependency on the patient inspiratory force, should be considered (1). Often, excipient particles lack the most adequate characteristics when obtained from primary manufacturing and have to be further engineered. Therefore, in the present work, we aim to explore innovative engineering strategies that allow the production of D-mannitol particles, which have adequate characteristics, to be successfully applied as carriers for DPI.
Wet-sieving, commonly used in soil analysis (11), and previously used by Adi et al. to fractionate lactose particles intended for inhalation (12), was selected to engineer D-mannitol. During wet-sieving, powder particles are washed with an anti-solvent and fractionated. Thus, wet-sieving was used for removing the variable amount of fine particles present in the raw excipient powder. Spray-congealing (also known as spray-chilling or spray-cooling), which is a melt-based technique able to produce well-defined spherical particles (13), was also applied to engineer D-mannitol. In this process, molten D-mannitol was atomized into droplets through a bi-fluid nozzle and sprayed into a cooling chamber where the melt quickly solidified forming solid microparticles. The technique was selected due to its known ability to produce free-flowing particles with a diameter between 50 and 500 μm.
To evaluate the impact of engineering on particle properties, D-mannitol powders were characterized concerning their solid-state, micromeritics and flowability. Afterwards, D-mannitol was blended (in binary and ternary mixtures) in low doses (1 wt%) with an inhaled corticosteroid (i.e., beclomethasone dipropionate). The obtained blends were tested in combination with a reservoir inhaler (NEXThaler®) and the aerosolization performance of the distinct particles of D-mannitol was evaluated, accordingly. Whenever relevant, the performance of the D-mannitol particles was compared to that of α-LH engineered by the same techniques. Eventually, it was possible to understand the applicability of wet-sieving and spray-congealing in producing novel D-mannitol carriers for DPI delivery.
Article information: Pinto, J.T., Zellnitz, S., Guidi, T. et al. Spray-Congealing and Wet-Sieving as Alternative Processes for Engineering of Inhalation Carrier Particles: Comparison of Surface Properties, Blending and In Vitro Performance. Pharm Res (2021). https://doi.org/10.1007/s11095-021-03061-5